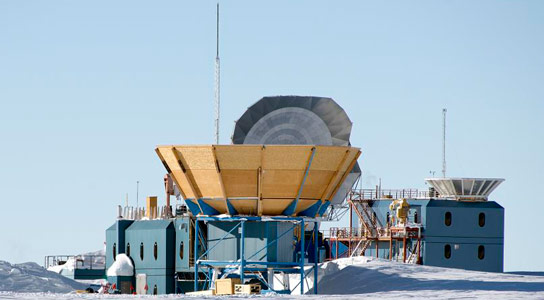
A photo of the Keck Array telescope at the South Pole, one of several facilities designed to study the cosmic microwave background. Credit: Harvard/Keck Array
An international team of scientists has published an extensive report on the future of astrophysical neutrino measurements, discussing how facilities that measure the microwave background will in the next decade also enable scientists to study the nature of neutrinos, and lead to fundamental insights into the basic laws of nature.
One of the most remarkable aspects of modern cosmology is that the properties of the largest physical structures in the universe reveal the properties of the smallest. The largest structures include the grand patterns seen in the cosmic microwave background radiation (CMBR) and the filamentary collections and nodes of clusters of galaxies in the early universe; the smallest include the elusive neutrinos. These hard-to-detect particles were for many decades thought to have no mass and to travel at the speed of light, like photons (quanta /particles of light). They are so hard to detect because they interact only very weakly with other kinds of matter, mostly passing through the matter unaffected.
During the past decades, scientists have discovered that there are three kinds of neutrinos and that in fact they all have some mass. The lightest kind is about a million times less massive than an electron. The reason light-weight neutrinos have such cosmological importance is that there are so many of them: The largest number of particles in the cosmos are CMBR photons, created in the later stages of the big bang, but the second largest number are neutrinos. Since the neutrinos have some slight mass while photons have none, their large number leads to a mass density (or equivalently, an energy density) today at least twenty-five times larger than that of the CMBR photons. The high density of these particles streaming past other cosmic matter without interacting with it tends to inhibit the growth of galactic structures (like those filamentary collections of galaxies). That tendency can be measured and quantified.
Neutrinos also impact two other major research areas. The first is the understanding of dark matter. Although neutrinos themselves are not dark matter, their total mass must be understood and accounted for to accurately study dark matter. The second is an improved understanding of the fundamental physics of elementary particles and forces. A better measurement of the masses of the three kinds of neutrinos would constrain the current models of physics. Since neutrinos interact so weakly with matter, however, Earth-bound measurements are extremely difficult to do, and as a result, the masses of neutrinos are only approximately known today, at roughly the 10% precision level. Cosmic measurements obtain neutrino masses by studying how they shape cosmic structures, and promise to be a better way to obtain more accurate and precise values.
An international collaboration of physicists and astronomers from around the world has published an extensive report on the future of astrophysical neutrino measurements. CfA astronomers Colin Bischoff and John Kovac were members of this group. The report summarizes several new experimental projects underway aimed at measuring the influence of cosmological neutrinos, with the expectation that in the next decade astronomy will be able to constrain the masses of the neutrinos to values about ten times more accurate and precise than today’s values – enough to indicate whether current particle physics is about right or needs to be replaced, and to clarify the nature of dark matter.
Reference: “Neutrino physics from the cosmic microwave background and large scale structure” by K. N. Abazajian, K. Arnold, J. Austermann, B. A. Benson, C. Bischoff, J. Bock, J. R. Bond, J. Borrill, E. Calabrese, J. E. Carlstrom, C. S. Carvalho, C. L. Chang, H. C. Chiang, S. Church, A. Cooray, T. M. Crawford, K. S. Dawson, S. Das, M. J. Devlin, M. Dobbs, S. Dodelson, O. Doré, J. Dunkley, J. Errard, A. Fraisse, J. Gallicchio, N. W. Halverson, S. Hanany, S. R. Hildebrandt, A. Hincks, R. Hlozek, G. Holder, W. L. Holzapfel, K. Honscheid, W. Hu, J. Hubmayr, K.Irwin, W. C. Jones, M. Kamionkowski, B. Keating, R. Keisler, L. Knox, E. Komatsu, J. Kovac, C.-L. Kuo, C. Lawrence, A. T. Lee, E. Leitch, E. Linder, P. Lubin, J. McMahon, A. Miller, L. Newburgh, M. D., Niemack, H. Nguyen, H. T. Nguyen, L. Page, C. Pryke, C. L. Reichard, J. E. Ruhl, N. Sehgal, U. Seljak, J. Sievers, E. Silverstein, A. Slosar, K. M. Smith, D. Spergel, S. T. Staggs, A. Stark, R. Stompor, A. G. Vieregg, G. Wang, S. Watson, E. J. Wollack, W. L. K. Wu, K. W. Yoon, O. Zahn, Topical Conveners: K. N. Abazajian, J. E. Carlstrom and A. T. Lee, 3 July 2014, Astroparticle Physics.
DOI: 10.1016/j.astropartphys.2014.05.014
Be the first to comment on "The Future of Astrophysical Neutrino Measurements"