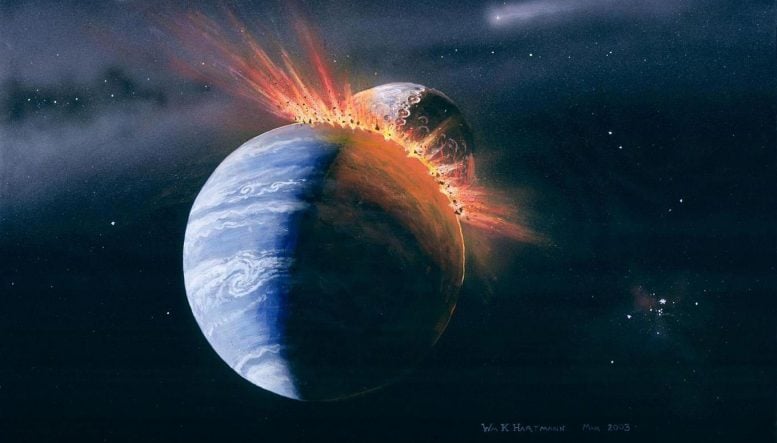
Scientists believe the moon formed after a large object crashed into the Earth, but details have been murky about what happened next. Credit: Illustration by William Hartmann
University of Chicago scientists measure rubidium isotopes to create model for moon formation.
Most people only ever encounter rubidium as the purple color in fireworks, but the obscure metal has helped two University of Chicago scientists propose a theory of how the moon may have formed.
Conducted in the lab of Prof. Nicolas Dauphas, whose pioneering research studies the isotopic makeup of rocks from Earth and the moon, the new study measured rubidium in both planetary bodies and created a new model to explain the differences. The breakthrough reveals new insights into a conundrum about the moon’s formation that has gripped the field of lunar science over the past decade, known as the “lunar isotopic crisis.”
This crisis kicked off when new methods of testing revealed Earth and moon rocks have strikingly similar levels of some isotopes, but very different levels of others. This confounds both major scenarios for how the moon formed: one being that a giant object smashed into Earth and took a chunk with it on its way to becoming the moon (in which case the moon should have a decisively different makeup, mostly the foreign object); and the other being that this object obliterated the Earth, and the two celestial bodies eventually formed out of the resulting smithereens (in which case the two makeups should be virtually identical).
“There’s clearly something missing there,” said Nicole Nie, PhD’19, first author of the study, recently published in Astrophysical Journal Letters. A former graduate student in Dauphas’ lab, Nie is now at the Carnegie Institution for Science.
To test different theories, Dauphas’ lab has a collection of moon rocks on loan from NASA, (representing every Apollo mission that recovered samples). Nie came up with a rigorous way to measure the isotopes of rubidium—an element that had never been precisely measured in moon rocks because it’s so difficult to isolate from potassium, which is chemically extremely similar.
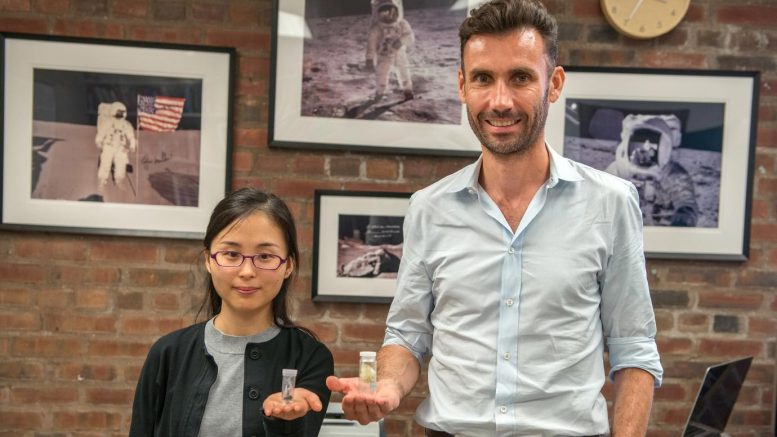
Professor Nicolas Dauphas (right) and Nicole Nie, PhD’19, use Apollo lunar rock samples to try to decode the age and composition of the moon. Credit: Photo by Joe Sterbenc
Rubidium is one of a family of elements that consistently shows up with different proportions of isotopes in the moon compared to the Earth. When Nie examined the moon rocks, she found they did in fact contain fewer of rubidium’s light isotopes and more heavy ones than Earth rocks do.
“There was really no framework for how this difference happened,” Dauphas said, a professor in the Department of Geophysical Sciences. “So we decided to make one.”
They started from the idea that both the Earth and the giant object were vaporized after the impact. In this scenario, a mass that will become Earth slowly coalesces, and an outer ring of debris forms around it. It’s still so hot, nearly 6,000 degrees Fahrenheit (3,300 degrees Celsius), that this ring is probably an airy outer layer of vapor surrounding a core of liquid magma.
Over time, Nie and Dauphas surmise, the lighter isotopes of elements like rubidium evaporate more readily. These condense onto the Earth, while the rest of the heavier isotopes left behind in the ring eventually form the moon.
This told them more about what the early moon and Earth would have looked like. Because they know exactly how much more of the lighter isotopes evaporated, they worked backward to find out how saturated the vapor layer would have been—the more saturated, the slower the evaporation. (Think of trying to dry out your laundry on a very humid day in the tropics, versus a dry day in the desert.)
“There was really no framework for how this difference happened. So we decided to make one.” — Prof. Nicolas Dauphas
This is helpful because the exact characteristics of this early phase have been hard to pin down. The results also fit nicely with previous measurements of other isotopes in moon rocks, such as potassium, copper, and zinc. “Our new scenario can quantitatively explain the lunar depletion of not only rubidium, but also most volatile elements,” Nie said.
The study is a long-needed step to connect the lines between isotope measurements and physical models of the proto-planetary bodies, Dauphas said.
“This was a link that was missing, and we hope it will help to constrain the scenarios for the early moon and Earth formation going forward,” he said.
Reference: “Vapor Drainage in the Protolunar Disk as the Cause for the Depletion in Volatile Elements of the Moon” by Nicole X. Nie and Nicolas Dauphas, 17 October 2019, The Astrophysical Journal Letters.
DOI: 10.3847/2041-8213/ab4a16
Be the first to comment on "New Analysis of NASA Moon Rocks Leads to Breakthrough on How the Earth and Moon Actually Formed"