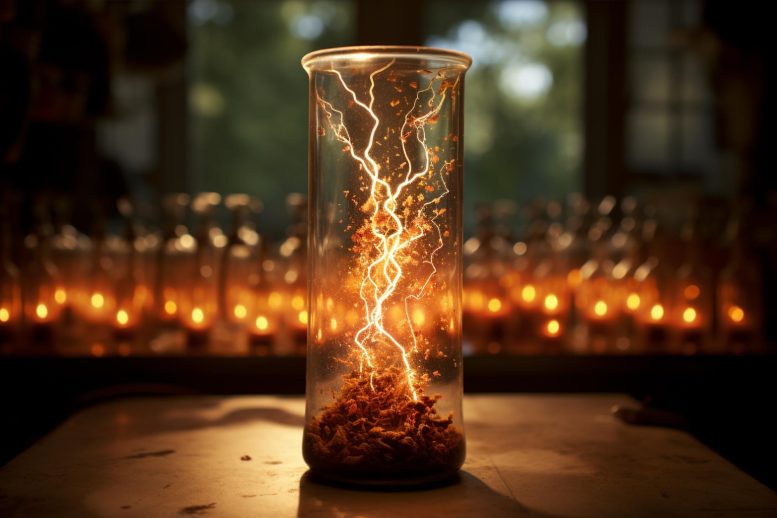
NASA scientists, along with a global team, are pushing the boundaries to understand how life might originate and evolve in diverse cosmic environments, both within our solar system and beyond.
Scientists are pushing to understand life’s chemical origins and potential development in cosmic environments, investigating scenarios like hydrothermal vents, meteorites, potential exotic life on Saturn’s moon, and lab-based Darwinian evolution. Research involves simulating hydrothermal vents, examining meteorites for life’s building blocks, and exploring Titan’s potential to support unfamiliar life forms. The efforts blend lab work and space exploration, pointing to a promising future in astrobiology.
Growing chemical gardens. Searching for life’s building blocks in meteorites. Sketching out a path to exotic life on a moon of Saturn.
Interplanetary probes and space telescopes take the search for life beyond Earth to new heights. But just as indispensable is the laboratory work on Earth itself. Experimenters seek to puzzle out the chemical origins of life, or capture evidence of molecules common to living things in samples from other objects in the solar system.
Darwinian Evolution in a Test Tube
Some even have kickstarted Darwinian evolution in a test tube. The process of natural selection, made famous by Charles Darwin, was seen in the well-known experiment, and appeared to meet, at least technically, NASA’s working definition of life: “a self-sustaining chemical system capable of Darwinian evolution.”
NASA scientists are part of a global push to understand how life began on our planet, how it might develop on others, and how we can use technology to detect it, within our solar system or in the stars beyond.
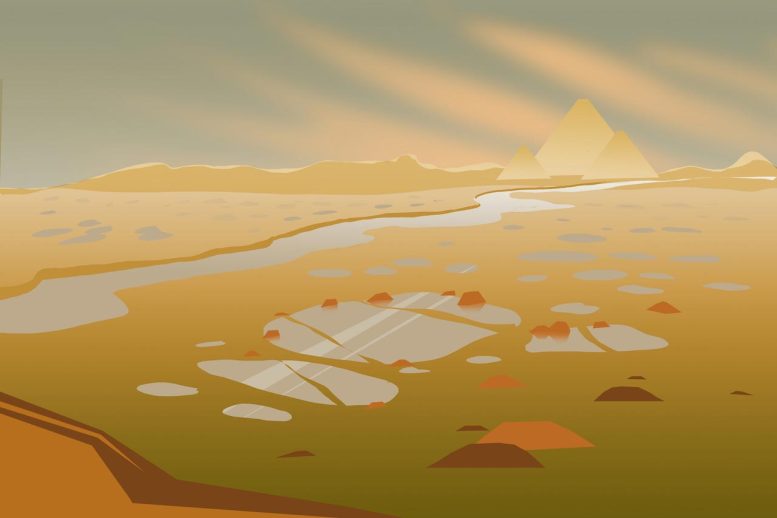
A bird’s eye view – though from a time perhaps 3.7 billion years ago when birds did not yet exist – shows Earth’s surface with a flowing river and vernal ponds, which could have played host to some of the earliest life-forms. Credit: NASA/JPL-Caltech/Lizbeth B. De La Torre
“My origin-of-life work is focused on how to get from a geochemical environment to the start of organic chemistry,” said Laurie Barge, co-leader of the Origins and Habitability Lab at NASA’s Jet Propulsion Laboratory in Southern California. “We don’t know what pieces of the first life came when. What came first? What came later? Was it before or after the cell emerged?”
Barge is known for chemical gardens – flasks full of materials that attempt to simulate the environment, chemistry, and even the electrical charge of hydrothermal vents on the floors of primordial oceans. They’re designed to explore how metabolism, a critical component of all life, might have chugged into operation in such vents some 4 billion years ago.
Astrobiological Theories and Hydrothermal Vents
Hydrothermal vents are just one of several scenarios astrobiologists have suggested as early paths to eventual life, and metabolism is not even life itself – just a way of turning organic compounds into energy, a baseline requirement for any living thing. The process later might have been co-opted by opportunistic, incipient life forms, though no one knows just how that could have happened.
These hydrothermal vents, or “chimneys,” also might be present on the sea floor of Saturn’s moon, Enceladus, or other “ocean worlds” that hide global oceans under shells of ice.
“These chimneys on the early Earth, also Enceladus: What types of environments, what sort of chemistry do those drive?” Barge asks. “What kind of energy do they generate?”
Whether the elements of eventual life began on a sea floor or, say, a pond on the land surface, they might have been infused with ingredients delivered from above.
Rocks as Time Travelers
While we can’t travel back in time to early Earth, many asteroids have remained unchanged for billions of years, making them akin to time capsules of the infant solar system. What’s more, pieces of space rocks that fall to Earth, called meteorites, also contain clues about the building blocks of early planets, and maybe even life.
At NASA’s Goddard Space Flight Center in Greenbelt, Maryland, Jason Dworkin, senior scientist for astrobiology, is investigating the composition and chemistry of meteorites.
Dworkin’s lab also analyzes samples of other solar system bodies returned to Earth by missions like Hayabusa2 from the Japanese space agency, JAXA, NASA’s Stardust, and Apollo, and soon-to-be-delivered asteroid samples from NASA’s OSIRIS-REx.
Organic compounds in rocks from space, though not signs of biology in themselves, might have been important to the origin of life on Earth – especially in the early period after Earth’s formation, when large asteroids were striking the surface more frequently.
“We try to understand the chemistry that could have been happening on Earth,” Dworkin said. “Though we know extraterrestrial material was raining down on Earth, we don’t know how important it was for life. We don’t know if it was a major or minor component, or the silver bullet that caused it to happen.”
Still, research by Dworkin and others has yielded potentially significant clues. In 2009, his lab was the first to detect an amino acid called glycine – a building block of life – in a comet, after analyzing samples returned by NASA’s Stardust spacecraft.
A more recent sample return in 2020, material from the rocky Ryugu asteroid delivered by Hayabusa2, provided evidence of amino acids, sulfur compounds, and even uracil, an important informational unit in the RNA found in Earth life, and niacin, a form of vitamin B3.
And OSIRIS-REx, now carrying a sample of material from the asteroid Bennu back to Earth, already has revealed veins of carbonate on the asteroid’s surface. The sample could shed light on the chemistry of such bodies, perhaps analogous to early chemistry on Earth.
Even the composition of asteroids could offer insight into the early formation of Earth and other planets.
The coming Artemis missions, taking humans back to the Moon, will include collection of samples by Artemis III; another planned “Sample Return” mission to Mars will gather cached sample tubes and bring them back to Earth.
“I love being able to look at a sample directly from an object, and interrogate that,” Dworkin said. “With Hayabusa2, OSIRIS-REx, Artemis III coming up, Mars sample-return coming up, there’s going to be so much to look at. We’ll do comparative geochemistry, comparative astrobiology, across different objects.”
Titan on Earth
Within the solar system or beyond, another intriguing – if perhaps less likely – possibility is sometimes called “life as we don’t know it:” life-forms based on unfamiliar molecular components, or using a solvent other than water. Saturn’s moon, Titan, often has been cited as a potential environment for such exotic forms.
Titan’s surface is in such a deep freeze that water is essentially rock. Yet the moon has a thick atmosphere, lakes, rivers, and precipitation – the only solar system body other than Earth with such a liquid cycle.
The lakes and rivers are composed of methane and ethane. Could some form of life thrive on these liquids, as Earth life does on water?
Laboratory work has provided some surprising clues. Titan’s extremely low surface temperatures – minus 290 degrees Fahrenheit (minus 179 Celsius) – make heat energy for chemistry hard to come by. And chemical reactions require liquid to act as a solvent, though methane has proven to be a poor one, ethane only marginally better, said Jonathan Lunine, an astronomy professor at Cornell University who has studied possible paths of chemistry on Titan’s surface.
“If you can’t get things dissolved in a liquid, not much is going to happen,” Lunine said.
But experiments by researchers at JPL showed that, at least under some conditions, organic material can condense out of these liquids. One possibility involves precipitation from these liquids of crystal structures. The structures could then act as templates to form similar crystals, in essence reproducing themselves.
“Maybe stuff like that would lead to something sort of lifelike,” said Robert Hodyss, a laboratory studies group supervisor at JPL.
The lab work is suggestive, he said, but much more data will be needed from Titan itself. That is expected to come from NASA’s Dragonfly spacecraft, a dual-quadcopter that will fly from site to site on Titan’s surface to conduct some “lab work” of its own. Dragonfly is expected to reach the smoggy moon in the mid-2030s after a seven-year journey.
“The Dragonfly mission is looking for prebiotic molecules that look familiar to us,” said Melissa Trainer at NASA Goddard, the deputy principal investigator for the mission. “We’re looking at the chemical inventory once we get there, to get an idea of relative abundances, the context in which we find them.”
That will allow investigators to interpret the findings, and perhaps determine whether the molecules and chemistry suggest the potential for life. “We could see an indication, with our best understanding, that could be relevant for some kind of biochemistry, even if it’s one that isn’t familiar,” she said.
Evidence of exotic life forms thriving on methane or ethane, however, might be difficult to detect, even if they somehow developed on Titan’s surface.
“Once you get into ‘life as we don’t know it,’ there are a lot of open questions there,” Trainer said.
Yet Titan – like Jupiter’s moon, Europa, or Saturn’s Enceladus – also is likely to be an ocean world, harboring liquid water beneath an ice-covered surface. That raises another possibility: organic material from the surface pushed deep into the moon, making contact with the subsurface ocean and, finding higher temperatures and pressures, interacting in ways that could produce a habitable environment.
Scientists also have proposed that surface impacts could drive organics from the surface into the underlying ocean.
Or, Trainer said, such an impact, or even volcanic eruptions of frozen material – “cryovolcanoes” – could create warm conditions allowing liquid water environments to thrive for a time. Lab work has shown that compounds containing carbon, hydrogen, and nitrogen that might be building blocks of biochemistry could accumulate on the surface under such conditions.
How Life Began
While such findings can yield key insights, astrobiology in the lab is not limited to creation of potentially habitable conditions, exotic or otherwise. Other experiments explore pathways to the start of life itself.
Among the best-known of these was conducted by Gerald Joyce, a research professor at the Salk Institute in La Jolla, California, and collaborator Tracey Lincoln. They created an RNA-based system, then coaxed it into sustained Darwinian evolution in a test tube. Lincoln, lead author and Joyce’s PhD student at the time, published the finding in 2009.
Although it technically met NASA’s working definition of life – a self-sustaining chemical system capable of Darwinian evolution – Joyce says that in his view, it still didn’t qualify as a true life form.
“I was the first to say, ‘It doesn’t make it,’” Joyce said. Left to its own, the system, with its fragile, low-capacity RNA molecule, would hardly be able to evolve from where it started.
“It wouldn’t have been too long till it was dead,” he said. “There was just not enough information carrying capacity” in the relatively short strands of RNA that were used.
In Joyce’s view, life’s system for recording and transmitting information must, in itself, possess enough information capacity to evolve entirely new processes – body armor, locomotion, or reproductive strategies, for example.
“We’re talking about more than just being ‘capable’ of undergoing Darwinian evolution,” he said. “It has to have – this is where it gets hard – some broad capacity to undergo Darwinian evolution. You need enough information to keep evolving: sensory systems, nervous systems, things like photosynthesis. If there’s enough complexity to invent a new biochemical function – that would do it for me.”
Still, his system might have borne at least a passing resemblance to some of the earliest forms of life. Research suggests that an “RNA” world might have preceded our DNA-dominated present. Although a more delicate molecule with far lower capacity to store information, RNA could have taken the first, halting steps toward life as we know it.
“There’s very good circumstantial evidence, based on the way biology unfolded, that RNA-based life preceded DNA,” Joyce said.
Concluding Thoughts
In the broad view, he said, momentum in astrobiology appears to be building. Advances in laboratory understanding of life’s components are beginning to converge with groundbreaking exploration of our solar system and deeper observation of planets around other stars.
“Many exciting things are happening,” he said. “There’s a focus on extrasolar planets, but what is happening on Mars is also incredible, with rovers, a helicopter, and a sample-return mission coming. And the next target will be the icy outer moons. It’s a really fun time in astrobiology.”
Search for Life
- Part 1: Beginnings: Life on Our World and Others
- Part 2: Life on Other Planets: What Is Life and What Does It Need?
- Part 3: The Hunt for Life on Mars and Elsewhere in the Solar System
- Part 4: Unraveling the Cosmic Origins of Life in the Lab
Be the first to comment on "Primordial Puzzles: Unraveling the Cosmic Origins of Life in the Lab"