A new study proposed a scheme to achieve ultrahigh reduced transition temperature, up to Tc/TF ~ 1, in two-component atomic Fermi gases, a system that mimic high Tc superconductors, via a tunable pairing interaction strength, using mixed dimensions where one component is in a deep one-dimensional (1D) optical lattice with a large lattice spacing, while the other remains in 3D free space. Quantum atomic Fermi gases have provided an ideal prototype for studying the physics of high Tc superconductivity, including the underlying pairing mechanism and the unusual pseudogap phenomena, which have been at the heart of debate in the field of high Tc superconductivity.
In a Letter published in SCIENCE CHINA Physics, Mechanics & Astronomy, theorists from Zhejiang University, Sun Yat-Sen University, and Zhejiang University of Technology, as well as the Synergetic Innovation Center of Quantum Information and Quantum Physics, report that by tuning the lattice spacing to be large in a mixed dimensional setting, the reduced superfluid transition temperature, Tc/TF, can be made extremely high, higher than that for any known systems.
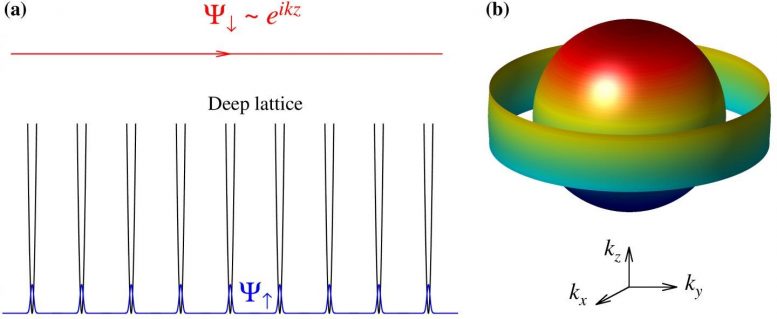
Figure 1. Fermionic atoms in mixed dimensions in the (a) real and (b) momentum space. Spin up atoms are subject to a 1D optical lattice in the z direction, while the spin down atoms in the 3D free space with a plane-wave wave function. The blue curve shows schematically the wavefunction of the spin up atoms. Spin up and down atoms occupy a thin disc and 3D sphere in momentum space, respectively. Credit: © Science China Press
It has been a long-term goal in the field of superconductivity to achieve a high transition temperature Tc, ideally up to room temperature (about 300 K or 27 C/80 F) and above. Given that the typical electron kinetic energy, represented by its Fermi temperature TF, is of the order of 10000 K (9,700 C or 17,500 F) in a solid, this is only a small fraction. Conventional superconductors in metals and alloys have a transition temperature of a few Kelvins, usually below 20 K (-250 C or -420 F). The high Tc cuprate superconductors, discovered in 1986, has a Tc up to 95 K (-180 C or -290 F) at the optimal oxygen doping concentration under ambient pressure, and up to 164 K (-110 C or -160 F) under high pressure, has a relatively low TF due to strong electron correlations, which pushes the reduced temperature Tc/TF up to about 0.05.
This ratio does not exceed this value for other families of superconductors, either, including iron-based superconductors, heavy fermion superconductors, organic superconductors, the monolayer FeSe/SrTiO3 superconductors, and the newly discovered magic angle twisted bilayer graphene, as well as the Tc record holder, H2S under high pressure. A higher ratio is made possible in ultracold atomic Fermi gases, with Tc/TF up to 0.218 in the BEC limit in the 3D homogeneous case. This ratio can be further raised to 0.518 in a harmonic trap in the BEC limit, utilizing the enhanced local Fermi energy at the trap center. This enhancement led to the idea of using mixed dimensions to tune the Fermi energy as a function of lattice spacing.
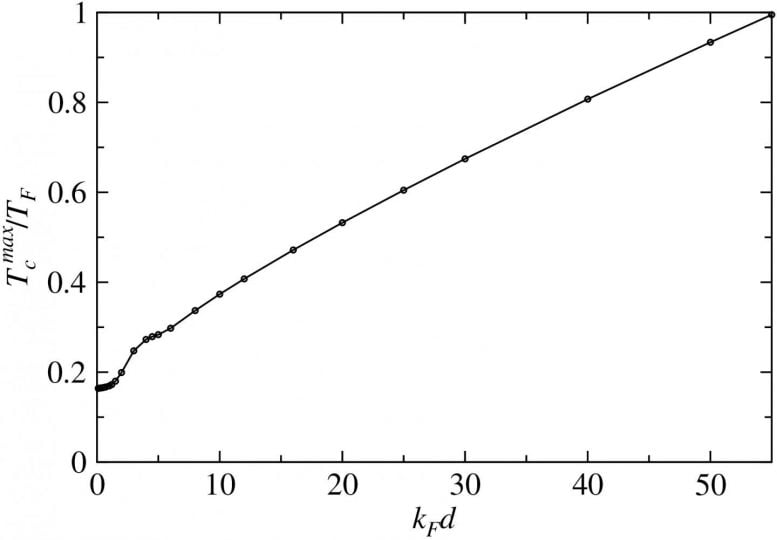
Figure 2. The ratio Tcmax/TF increases roughly linearly as a function of kFd, where Tcmax is the maximum Tc for given lattice constant d as the pairing strength varies. Credit: © Science China Press
The idea of enhancing Tc/TF via mixed dimensions is illustrated in Figure 1. One of the two pairing components, referred to as spin down atoms, remain in 3D free space, occupying an isotropic Fermi sphere in momentum space. On the contrary, the spin up atoms are subject to a deep 1D optical lattice potential (in the z direction) with a large band gap and a large lattice spacing d so that the fermions occupy a thin disc of thickness 2p/d and radius kF so that the Fermi energy EF for the spin up atoms increases with increasing d. The lattice may be so deep that the spin-up atoms are essentially localized in their respective lattice sites in the absence of pairing interaction.
Then, when the pairing interaction is turned on and tuned to be large via Feshbach resonance, the Fermi sphere of the spin down atoms will be deformed into a disc to match that of the spin up atoms. As a result, both components now acquire a large Fermi energy (in comparison with the non-interacting 3D value, EF) and thus led to a high Tc/TF.
As pointed out by the authors, despite that the spin up atoms are highly localized in the absence of pairing interaction, the atomic pairs acquire high mobility due to the spin down components. This is somewhat similar to the case of superconductivity in a flat band, where individual electrons are localized whereas Cooper pairs acquire mobility via the pairing interaction. The enhancement of Tc/TF is largely governed by the ratio EF /EF. The result is shown in Figure 2, where Tcmax is the maximum Tc as a function of pairing strength for a given lattice spacing d. The ratio reaches about unity for kFd = 55, higher than any known systems. (Note here EF=KBTF=2K2F/2m, where kB is the Boltzmann constant, ? the Planck constant h divided by 2p, m the mass of atoms, and kF and EF the Fermi momentum and Fermi energy, respectively, for the system in the absence of lattice potential and pairing interaction.)
As emphasized by the authors, the above idea of enhancing Tc/TF is independent of specific details of their theory and works equally well for rival theories. The proposed scheme may be realized experimentally with isotopic atoms such as 161Dy and 163Dy, once a proper Feshbach resonance is identified.
Ultracold atomic systems have been widely studied for their capability of quantum simulation and quantum engineering. An important goal of the atomic Fermi gas community is to simulate and help to understand the mechanism of high Tc superconductivity. A proper understanding will certainly be critical in the search for and design of new superconductors of higher Tc.
Reference: “Ultra high temperature superfluidity in ultracold atomic Fermi gases with mixed dimensionality” by Leifeng Zhang, Jibiao Wang, Yi Yu and Qijin Chen, 2 September 2019, Science China Physics, Mechanics & Astronomy.
DOI: 10.1007/s11433-019-9452-y
This research was funded by the National Natural Science Foundation of China (Grant Nos. 11274267, and 11774309), the National Basic Research Program of China (Grant No. 2012CB927404), and the Natural Science Foundation of Zhejiang Province of China (Grant No. LZ13A040001).
Be the first to comment on "Ultra High Temperature Superfluidity in Ultra Cold Atomic Gases via Mixed Dimensions"