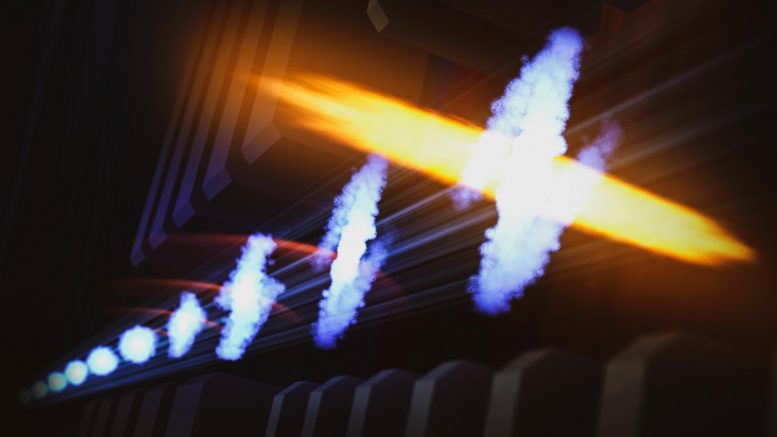
A SLAC-led team has invented a method, called XLEAP, that generates powerful low-energy X-ray laser pulses that are only 280 attoseconds, or billionths of a billionth of a second, long and that can reveal for the first time the fastest motions of electrons that drive chemistry. This illustration shows how the scientists use a series of magnets to transform an electron bunch (blue shape at left) at SLAC’s Linac Coherent Light Source into a narrow current spike (blue shape at right), which then produces a very intense attosecond X-ray flash (yellow). Credit: Greg Stewart/SLAC National Accelerator Laboratory
Less than a millionth of a billionth of a second long, attosecond X-ray pulses allow researchers to peer deep inside molecules and follow electrons as they zip around and ultimately initiate chemical reactions.
Scientists at the Department of Energy’s SLAC National Accelerator Laboratory devised a method to generate X-ray laser bursts lasting hundreds of attoseconds (or billionths of a billionth of a second) in 2018. This technique, known as X-ray laser-enhanced attosecond pulse generation (XLEAP), enables researchers to investigate how electrons racing about molecules initiate key processes in biology, chemistry, materials science, and other fields.
“Electron motion is an important process by which nature can move energy around,” says SLAC scientist James Cryan. “A charge is created in one part of a molecule and it transfers to another part of the molecule, potentially kicking off a chemical reaction. It’s an important piece of the puzzle when you start to think about photovoltaic devices for artificial photosynthesis, or charge transfer inside a molecule.”
Now, researchers at SLAC’s Linac Coherent Light Source (LCLS) have rattled the electrons in a molecule using attosecond pulses to create an excited quantum state and measure how the electrons behave in this state in never-before-seen detail. The findings were recently published in the journal Science.
“XLEAP allows us to peer deep inside molecules and follow electron motion on its natural time scale,” says SLAC scientist Agostino Marinelli, who leads the XLEAP project. “This could provide insight into many important quantum mechanical phenomena, where electrons typically play a key role.”
Electronic messengers
Attosecond pulses are the shortest pulses generated at X-ray free-electron lasers like LCLS. The unique achievement of the XLEAP project has been to make attosecond pulses at the right wavelength to look inside the most important small atoms, such as carbon, nitrogen and oxygen. Like cameras with ultrafast shutter speeds, XLEAP pulses can capture the movements of electrons and other motions on an extremely fast timescale that could not be resolved before.
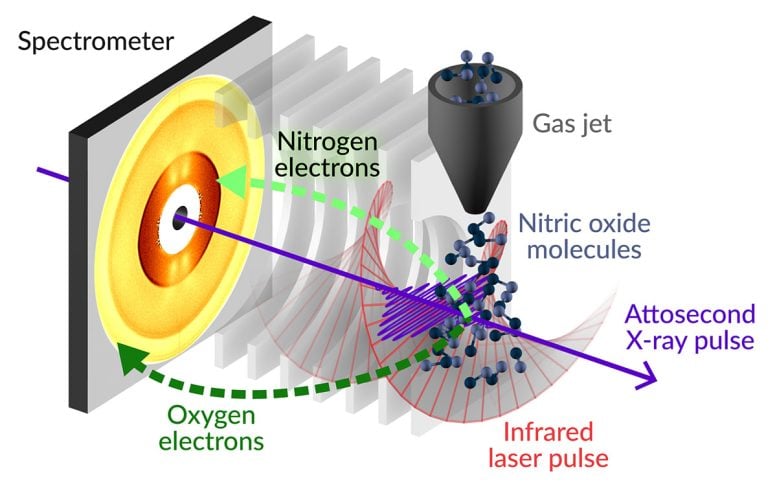
In this experiment, the researchers hit nitric oxide molecules with an X-ray pulse, knocking electrons out of their normal position and into a highly excited electron cloud. They created an ultrafast clock with a circularly polarized laser to measure what happened next. The electron cloud decayed by spitting out fast electrons, which were whirled around by the laser field before landing on the detector. The position in which the electrons landed on the detector helped the researchers figure out how the electron cloud was changing. They saw the cloud move in a unique quantum manner over the course of only a few millionths of a billionth of a second. Credit: Greg Stewart/SLAC National Accelerator Laboratory
When X-ray pulses interact with matter, they can boost some of the most tightly bound core electrons in the sample to highly energetic states, known as core-excited states. Because they are so energetic, core-excited states are extremely unstable and will typically decay very quickly by releasing energy in the form of a fast electron, known as an Auger-Meitner electron. This phenomenon has historically been known as Auger decay but recently scientists have chosen to add the name of Lise Meitner, who first observed the phenomenon, in recognition of her broad-ranging contributions to modern atomic physics.
In their study, the researchers precisely tuned the wavelength of the X-rays from LCLS to create a quantum state of matter called a coherent superposition, a manifestation of the wavelike nature of matter. Similar to Schrödinger’s cat, which found itself both dead and alive at the same time, the excited electrons were simultaneously in different core-excited states. This meant they were orbiting the molecule along different trajectories at the same time.
To follow how this coherent superposition of core-excited states unfolded over time, the researchers created an ultrafast clock known as an ‘attoclock,’ where a rapidly rotating electric field from a circularly polarized laser pulse acts as the clock hand. The Auger-Meitner electrons released in the decay of the core-excited states were whirled around by the circularly polarized laser pulse before landing on the detector. The position in which an electron landed on the detector told the researchers the time at which it was ejected from the molecule. By measuring the ejection times of many Auger-Meitner electrons, the researchers were able to build up a picture of how the coherent superposition state was changing with a time resolution of just a few hundred attoseconds.
“It’s the first time that we’re able to track this particular phenomenon and directly measure the rate of electron emission,” says SLAC scientist and lead author Siqi Li. “Our technique takes us a step beyond just seeing the process happen and allows us to spy on the intricate electron behavior happening in the molecule within a few millionths of a billionth of a second. It gives us a really nice way to look inside the molecule and see what’s happening on a very fast timescale.”
World-leading capability
To follow up on this experiment, the researchers are working on new measurements of more complex quantum behavior.
“In this experiment we are looking at the electronic behavior of a very simple model that you can almost solve with a pencil and paper,” says SLAC scientist and joint lead author Taran Driver. “Now that we’ve shown we can make these ultrafast measurements, the next step is to look at more complicated phenomena that theories are not yet able to accurately describe.”
The ability to make measurements on faster and faster timescales is exciting, Cryan says, because the first things that happen in a chemical reaction might hold the key to understanding what happens later.
“This research is the first time-resolved application of these ultrashort X-ray pulses, bringing us one step closer to doing really cool things like watching quantum phenomena evolve in real time,” he says. “It has the promise to become a world-leading capability that many people will be interested in for years to come.”
LCLS is a DOE Office of Science user facility. This research is part of a collaboration between researchers from SLAC, Stanford University, Imperial College London and other institutions. It was supported by the Office of Science.
Reference: “Attosecond coherent electron motion in Auger-Meitner decay” by Siqi Li, Taran Driver, Philipp Rosenberger, Elio G. Champenois, Joseph Duris, Andre Al-Haddad, Vitali Averbukh, Jonathan C. T. Barnard, Nora Berrah, Christoph Bostedt, Philip H. Bucksbaum, Ryan N. Coffee, Louis F. DiMauro, Li Fang, Douglas Garratt, Averell Gatton, Zhaoheng Guo, Gregor Hartmann, Daniel Haxton, Wolfram Helml, Zhirong Huang, Aaron C. LaForge, Andrei Kamalov, Jonas Knurr, Ming-Fu Lin, Alberto A. Lutman, James P. MacArthur, Jon P. Marangos, Megan Nantel, Adi Natan, Razib Obaid, Jordan T. O’Neal, Niranjan H. Shivaram, Aviad Schori, Peter Walter, Anna Li Wang, Thomas J. A. Wolf, Zhen Zhang, Matthias F. Kling, Agostino Marinelli and James P. Cryan, 6 January 2022, Science.
DOI: 10.1126/science.abj2096
I feel I can understand quantum mechanics if granted an opportunity