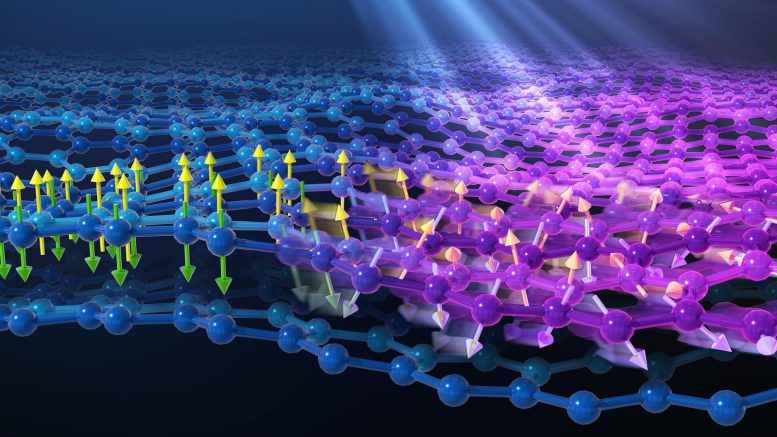
Atomic carpet moved by scrambled spins. Shearing of atomic layers in layered iron phosphorus trisulfide is caused by scrambling of electron spin upon exposure to light pulse. Ordered spins on left; scrambled spins on right. Credit: Image by Argonne National Laboratory
Cutting-edge ultrafast imaging techniques have revealed ultrafast mechanical motion tied to a change in magnetic state in a layered material. This intriguing magnetic effect could have applications in nanodevices requiring ultra-precise and fast motion control.
A common metal paper clip will stick to a magnet. Scientists classify such iron-containing materials as ferromagnets. A little over a century ago, physicists Albert Einstein and Wander de Haas reported a surprising effect with a ferromagnet. They found that when you suspend an iron cylinder from a wire and expose it to a magnetic field, it starts rotating if the direction of the magnetic field is reversed.
“Einstein and de Haas’s experiment is almost like a magic show,” said Haidan Wen, a physicist in the Materials Science and X-ray Science divisions of the U.S. Department of Energy’s (DOE) Argonne National Laboratory. “You can cause a cylinder to rotate without ever touching it.”
“In this experiment, a microscopic property, electron spin, is exploited to elicit a mechanical response in a cylinder, a macroscopic object.”
— Alfred Zong, Miller Research Fellow at the University of California, Berkeley
In the scientific journal Nature, a team of researchers from Argonne and other U.S. national laboratories and universities now report an analogous yet different effect in an “anti”-ferromagnet. This could have important applications in devices requiring ultra-precise and ultrafast motion control. One example is high-speed nanomotors for biomedical applications, such as use in nanorobots for minimally invasive diagnosis and surgery.
Electron Spin and Its Role
The difference between a ferromagnet and antiferromagnet has to do with a property called electron spin. This spin has a direction. Scientists represent the direction with an arrow, which can point up or down or any direction in between. In the magnetized ferromagnet mentioned above, the arrows associated with all the electrons in the iron atoms can point in the same direction, say, up. Reversing the magnetic field reverses the direction of the electron spins. So, all arrows are pointing down. This reversal leads to the cylinder’s rotation.
“In this experiment, a microscopic property, electron spin, is exploited to elicit a mechanical response in a cylinder, a macroscopic object,” said Alfred Zong, a Miller Research Fellow at the University of California, Berkeley.
Antiferromagnet Experiment
In antiferromagnets, instead of the electron spins all pointing up, for example, they alternate from up to down between adjacent electrons. These opposite spins cancel each other out, and antiferromagnets thus do not respond to changes in a magnetic field as ferromagnets do.
“The question we asked ourselves is, can electron spin elicit a response in an antiferromagnet that is different but similar in spirit to that from the cylinder rotation in the Einstein-de Hass experiment?” Wen said.
To answer that question, the team prepared a sample of iron phosphorus trisulfide (FePS3), an antiferromagnet. The sample consisted of multiple layers of FePS3, with each layer being only a few atoms thick.
“Unlike a traditional magnet, FePS3 is special because it is formed in a layered structure, in which the interaction between the layers is extremely weak,” said Xiaodong Xu, professor of physics and materials science at the University of Washington.
Result of Experiment
“We designed a set of corroborative experiments in which we shot ultrafast laser pulses at this layered material and measured the resultant changes in material properties with optical, X-ray, and electron pulses,” Wen added.
The team found that the pulses change the magnetic property of the material by scrambling the ordered orientation of electron spins. The arrows for electron spin no longer alternate between up and down in an orderly fashion, but are disordered.
“This scrambling in electron spin leads to a mechanical response across the entire sample. Because the interaction between layers is weak, one layer of the sample is able to slide back and forth with respect to an adjacent layer,” explained Nuh Gedik, professor of physics at the Massachusetts Institute of Technology (MIT).
This motion is ultrafast, at an incredible 10 to 100 picoseconds per oscillation. One picosecond equals just one trillionth of a second. This is so fast that in one picosecond, light travels a mere third of a millimeter.
Measurements on samples with spatial resolution on the atomic scale and temporal resolution measured in picoseconds require world-class scientific facilities. To that end, the team relied on cutting-edge ultrafast probes that use electron and X-ray beams for analyses of atomic structures.
Motivated by optical measurements at the University of Washington, the initial studies employed the mega-electronvolt ultrafast electron diffraction facility at SLAC National Accelerator Laboratory. Further studies were performed at an ultrafast electron diffraction setup at MIT. These results were complemented by work at the ultrafast electron microscope facility in the Center for Nanoscale Materials (CNM) and the 11-BM and 7-ID beamlines at the Advanced Photon Source (APS). Both CNM and APS are DOE Office of Science user facilities at Argonne National Laboratory.
Implications of the Discovery
The electron spin in a layered antiferromagnet also has an effect at longer times than picoseconds. In an earlier study using APS and CNM facilities, members of the team observed that fluctuating motions of the layers slowed down dramatically near the transition from disordered to ordered behavior for the electron spins.
“The pivotal discovery in our current research was finding a link between electron spin and atomic motion that is special to the layered structure of this antiferromagnet,” Zong said. “And because this link manifests at such short time and tiny length scales, we envision that the ability to control this motion by changing the magnetic field or, alternatively, by applying a tiny strain will have important implications for nanoscale devices.”
Reference: “Spin-mediated shear oscillators in a van der Waals antiferromagnet” by Alfred Zong, Qi Zhang, Faran Zhou, Yifan Su, Kyle Hwangbo, Xiaozhe Shen, Qianni Jiang, Haihua Liu, Thomas E. Gage, Donald A. Walko, Michael E. Kozina, Duan Luo, Alexander H. Reid, Jie Yang, Suji Park, Saul H. Lapidus, Jiun-Haw Chu, Ilke Arslan, Xijie Wang, Di Xiao, Xiaodong Xu, Nuh Gedik and Haidan Wen, 2 August 2023, Nature.
DOI: 10.1038/s41586-023-06279-y
Besides Wen, Zong, Xu, and Gedik, other authors include Qi Zhang, Faran Zhou, Yifan Su, Kyle Hwangbo, Xiaozhe Shen, Qianni Jiang, Haihua Liu, Thomas Gage, Donald Walko, Michael E. Kozina, Duan Luo, Alexander Reid, Jie Yang, Suji Park, Saul Lapidus, Jiun-Haw Chu, Ilke Arslan, Xijie Wang and Di Xiao.
This work was primarily supported by the DOE Office of Basic Energy Sciences.
As a scholar, have you ever asked yourself: Where does the power of electrons and celestial body spin come from? Will mathematics inspire you? Can topological vortices not spin?