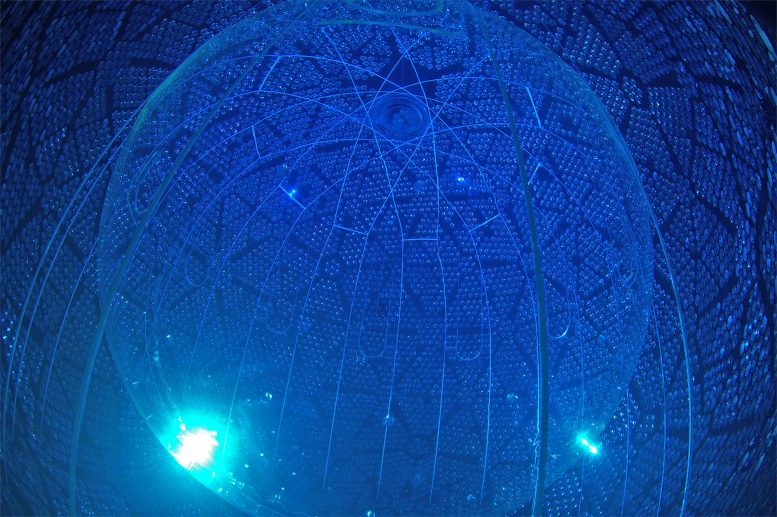
A view inside the SNO detector when filled with water. In the background, there are 9,000 photomultiplier tubes that detect photons and the acrylic vessel that (now) holds liquid scintillator. The ropes that crisscross on the outside hold it down when the scintillator is added to prevent it from floating upwards. The acrylic vessel is 12 m wide, about half the width of an Olympic-sized swimming pool. The detector is located in SNOLAB, a research facility located 2km underground near Sudbury, Canada. Credit: SNO+ Collaboration)
An international team of scientists has made a breakthrough in detecting neutrinos using pure water instead of the expensive liquid scintillator that was previously used. The Sudbury Neutrino Observation (SNO+) experiment, located in a mine in Sudbury, Ontario, detected subatomic particles, known as antineutrinos, using pure water. Neutrinos and antineutrinos are tiny subatomic particles that are considered fundamental building blocks of matter and have practical applications such as monitoring nuclear reactors and detecting nuclear activities. The researchers hope that an array of large and inexpensive reactors could be built to ensure that countries are adhering to nuclear weapons treaties.
Research published in the journal Physical Review Letters conducted by an international team of scientists including Joshua Klein, the Edmund J. and Louise W. Kahn Term Professor in the University of Pennsylvania School of Arts & Sciences, has resulted in a significant breakthrough in detecting neutrinos.
The international collaborative experiment known as Sudbury Neutrino Observation (SNO+), located in a mine in Sudbury, Ontario, roughly 240km (about 149.13 mi) from the nearest nuclear reactor, has detected subatomic particles, known as antineutrinos, using pure water. Klein notes that prior experiments have done this with a liquid scintillator, an oil-like medium that produces a lot of light when charged particles like electrons or protons pass through it.
“Given that the detector needs to be 240km, about half the length of New York state, away from the reactor, large amounts of scintillator are needed, which can be very expensive,” Klein says. “So, our work shows that very large detectors could be built to do this with just water.”
What neutrinos and antineutrinos are and why you should care
Klein explains that neutrinos and antineutrinos are tiny subatomic particles that are the most abundant particles in the universe and considered fundamental building blocks of matter, but scientists have had difficulty detecting them due to their sparse interactions with other matter and because they cannot be shielded, meaning they can pass through any and everything. But that doesn’t mean they’re harmful or radioactive: Nearly 100 trillion neutrinos pass through our bodies every second without notice.
These properties, however, also make these elusive particles useful for understanding a range of physical phenomena, such as the formation of the universe and the study of distant astronomical objects, and they “have practical applications as they can be used to monitor nuclear reactors and potentially detect the clandestine nuclear activities,” Klein says.
Where they come from
While neutrinos are typically produced by high energy reactions like nuclear reactions in stars, such as the fusion of hydrogen into helium in the sun wherein protons and other particles collide and release neutrinos as a byproduct, antineutrinos, Klein says, are usually produced artificially, “for instance, nuclear reactors, which, to split atomic nuclei, produce antineutrinos as a result of radioactive beta decay from the reaction,” he says. “As such, nuclear reactors produce large amounts of antineutrinos and make them an ideal source for studying them.”
Why this latest finding is a breakthrough
“So, monitoring reactors by measuring their antineutrinos tells us whether they are on or off,” Klein says, “and perhaps even what nuclear fuel they are burning.”
Klein explains that a reactor in a foreign country could therefore be monitored to see if that country is switching from a power-generating reactor to one that is making weapons-grade material. Making the assessment with water alone means an array of large but inexpensive reactors could be built to ensure that a country is adhering to its commitments in a nuclear weapons treaty, for example; it is a handle on ensuring nuclear nonproliferation.
Why this hasn’t been done before
“Reactor antineutrinos are very low in energy, and thus a detector must be very clean from even trace amounts of radioactivity,” Klein says. “In addition, the detector must be able to ‘trigger’ at a low enough threshold that the events can be detected.”
He says that, for a reactor as far away as 240km, it’s particularly important that the reactor contain at least 1,000 tons of water. SNO+ satisfied all these criteria.
Leading the charge
Klein credits his former trainees Tanner Kaptanglu and Logan Lebanowski for spearheading this effort. While the idea for this measurement formed part of Kaptanglu’s doctoral thesis, Lebanowski, a former postdoctoral researcher, oversaw the operation.
“With our instrumentation group here, we designed and built all the data acquisition electronics and developed the detector ‘trigger’ system, which is what allowed SNO+ to have an energy threshold low enough to detect the reactor antineutrinos.”
Reference: “Evidence of Antineutrinos from Distant Reactors Using Pure Water at SNO+” by A. Allega et al. (The SNO+ Collaboration), 1 March 2023, Physical Review Letters.
DOI: 10.1103/PhysRevLett.130.091801
Joshua Klein is the Edmund J. and Louise W. Kahn Professor and graduate chair in the Department of Physics & Astronomy in the University of Pennsylvania School of Arts & Sciences.
Capital construction funds for the SNO+ experiment were provided by the Canada Foundation for Innovation (CFI) and matching partners. SNOLAB operations are supported by the CFI and the Province of Ontario Ministry of Research and Innovation, with underground access provided by Vale at the Creighton mine site.
The research was funded by the Department of Energy Office of Nuclear Physics, the National Science Foundation, and the Department of Energy National Nuclear Security Administration through the Nuclear Science and Security Consortium.
This is the poorest science writing I’ve read, article is terrible