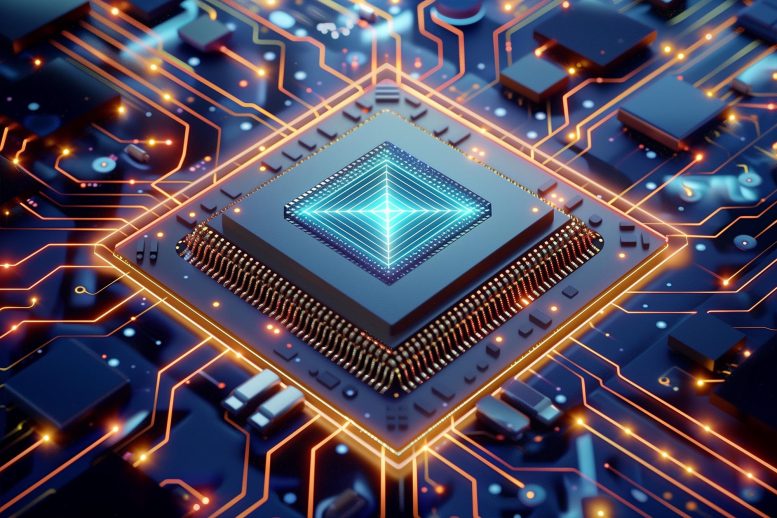
New microcapacitor technology developed at Berkeley Lab enhances energy storage capabilities on microchips, marking a major advancement in microelectronics. Credit: SciTechDaily
New microcapacitors developed by scientists show record energy and power densities, paving the way for on-chip energy storage in electronic devices.
Researchers are striving to make electronic devices smaller and more energy-efficient by integrating energy storage directly onto microchips. This approach minimizes the energy losses that occur when power is transferred between the different device components. In order to be effective, on-chip energy storage must be capable of storing a substantial amount of energy in a compact space and delivering it rapidly. Existing technologies, however, cannot meet these requirements.
Breakthrough in Microcapacitors
Scientists at Lawrence Berkeley National Laboratory (Berkeley Lab) and UC Berkeley have made a significant step towards overcoming these challenges, recently achieving record-high energy and power densities in microcapacitors. These capacitors are made from engineered thin films of hafnium oxide and zirconium oxide, employing materials and fabrication techniques common in chip manufacturing. Published in the journal Nature, their findings could revolutionize on-chip energy storage and power delivery in next-generation electronics.
“We’ve shown that it’s possible to store a lot of energy in microcapacitors made from engineered thin films, much more than what is possible with ordinary dielectrics,” stated Sayeef Salahuddin, a senior scientist at Berkeley Lab, UC Berkeley professor, and project lead. “What’s more, we’re doing this with a material that can be processed directly on top of microprocessors.” This research is part of broader efforts at Berkeley Lab to develop new materials and techniques for more efficient microelectronics.
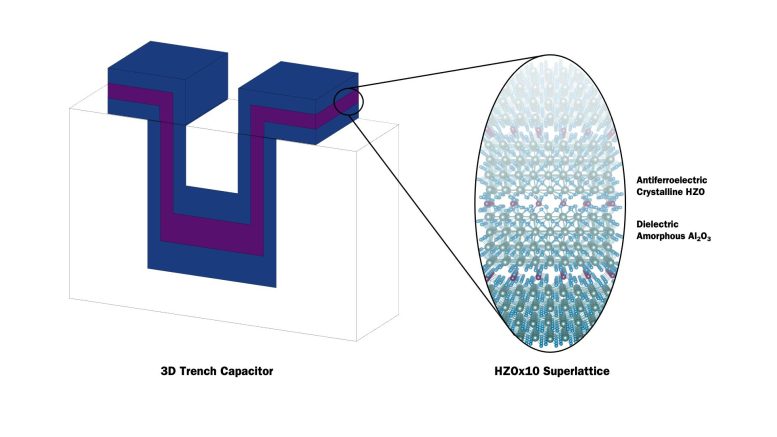
Microcapacitors made with engineered hafnium oxide/zirconium oxide films in 3D trench capacitor structures — the same structures used in modern microelectronics — achieve record-high energy storage and power density, paving the way for on-chip energy storage. Credit: Nirmaan Shanker/Suraj Cheema
Capacitor Fundamentals and Challenges
Capacitors are one of the basic components of electrical circuits but they can also be used to store energy. Unlike batteries, which store energy through electrochemical reactions, capacitors store energy in an electric field established between two metallic plates separated by a dielectric material. Capacitors can be discharged very rapidly when needed, allowing them to deliver power quickly. Also, they do not degrade with repeated charge-discharge cycles, giving them much longer lifespans than batteries. However, capacitors generally have much lower energy densities than batteries, meaning they can store less energy per unit volume or weight, and that problem only gets worse when you try to shrink them down to microcapacitor size for on-chip energy storage.
Research Methodologies and Results
The researchers created their revolutionary microcapacitors by carefully engineering thin films of HfO2-ZrO2 to achieve a negative capacitance effect. Normally, layering one dielectric material on top of another results in an overall lower capacitance. However, if one of those layers is a negative-capacitance material, then the overall capacitance actually increases. In earlier work, Salahuddin and colleagues demonstrated the use of negative capacitance materials to produce transistors that can be operated at substantially lower voltages than conventional MOSFET transistors. Here, they harnessed negative capacitance to produce capacitors capable of storing greater amounts of charge, and therefore energy.
The films are made from a mix of HfO2 and ZrO2 grown by atomic layer deposition, using standard materials and techniques from industrial chip fabrication. Depending on the ratio of the two components, the films can be ferroelectric, where the crystal structure has a built-in electric polarization, or antiferroelectric, where the structure can be nudged into a polar state by applying an electric field. When the composition is tuned just right, the electric field created by charging the capacitor balances the films at the tipping point between ferroelectric and antiferroelectric order, and this instability gives rise to the negative capacitance effect where the material can be easily polarized by even a small electric field.
“That unit cell really wants to be polarized during the phase transition, which helps produce extra charge in response to an electric field,” said Suraj Cheema, a postdoc in Salahuddin’s group and one of the lead authors of the paper. “This phenomena is one example of a negative capacitance effect but you can think of it as a way of capturing way more charge than you normally would have,” added Nirmaan Shanker, a graduate student in Salahuddin’s group, co-lead author.
To scale up the energy storage capability of the films, the team needed to increase the film thickness without allowing it to relax out of the frustrated antiferroelectric-ferroelectric state. They found that by interspersing atomically thin layers of aluminum oxide after every few layers of HfO2-ZrO2, they could grow the films up to 100 nm thick while retaining the desired properties.
Finally, working with collaborators at the MIT Lincoln Laboratory, the researchers integrated the films into three-dimensional microcapacitor structures, growing the precisely layered films in deep trenches cut into silicon with aspect ratios up to 100:1. These 3D trench capacitor structures are used in today’s DRAM capacitors and can achieve much higher capacitance per unit footprint compared to planar capacitors, allowing greater miniaturization and design flexibility. The properties of the resulting devices are record-breaking: compared to the best electrostatic capacitors today, these microcapacitors have nine times higher energy density and 170 times higher power density (80 mJ-cm-2 and 300 kW-cm-2, respectively).
“The energy and power density we got are much higher than we expected,” said Salahuddin. “We’ve been developing negative capacitance materials for many years, but these results were quite surprising.”
Future Directions
These high-performance microcapacitors could help meet the growing demand for efficient, miniaturized energy storage in microdevices such as Internet-of-Things sensors, edge computing systems, and artificial intelligence processors. The researchers are now working on scaling up the technology and integrating it into full-size microchips, as well as pushing the fundamental materials science forward to improve the negative capacitance of these films even more.
“With this technology, we can finally start to realize energy storage and power delivery seamlessly integrated on-chip in very small sizes,” said Cheema. “It can open up a new realm of energy technologies for microelectronics.”
Reference: “Giant energy storage and power density negative capacitance superlattices” by Suraj S. Cheema, Nirmaan Shanker, Shang-Lin Hsu, Joseph Schaadt, Nathan M. Ellis, Matthew Cook, Ravi Rastogi, Robert C. N. Pilawa-Podgurski, Jim Ciston, Mohamed Mohamed and Sayeef Salahuddin, 9 April 2024, Nature.
DOI: 10.1038/s41586-024-07365-5
Parts of this work were conducted at the Molecular Foundry, a DOE Office of Science nanoscience user facility located at Berkeley Lab. The research received support from the Department of Energy’s Office of Science, Office of Basic Energy Sciences, the Defense Threat Reduction Agency (DTRA), and the Secretary of Defense for Research and Engineering.
Be the first to comment on "Tiny Titans: Revolutionary Microcapacitors Set to Supercharge Next-Gen Electronics"