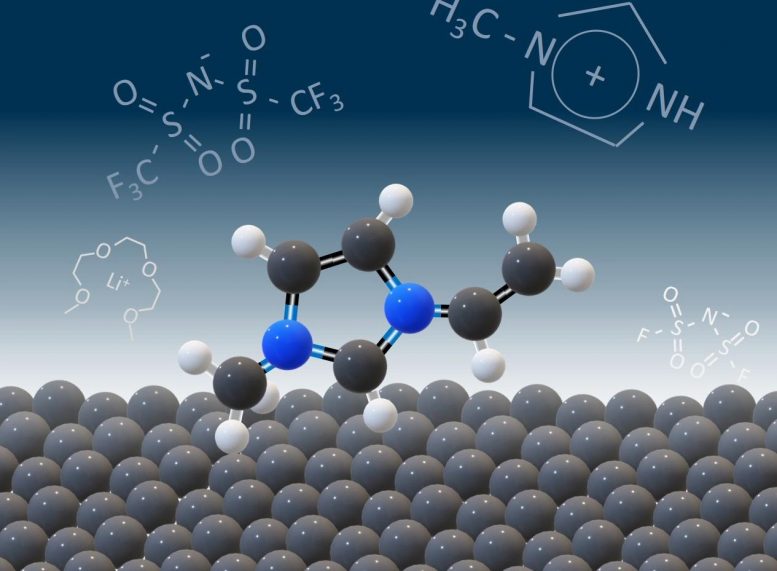
A forward-looking review encourages scientists to study electrode-ionic liquid coupling, which occurs at the interface of electrodes and electrolytes, when developing safer, more stable, and efficient energy storage devices. Credit: Xuehang Wang/Drexel University
Scientists seeking ways to improve a battery’s ability to hold a charge longer, using advanced materials that are safe, stable, and efficient, have determined that the materials themselves are only part of the solution.
In fact, studies at the interface of battery materials, along with increased knowledge of the processes at work, are unleashing a surge of knowledge needed to more quickly address the demand for longer-lasting portable electronics, electric vehicles, and stationary energy storage for the electric grid.
“If we need better energy storage, we need to better understand what happens at the interface between the electrolyte and the battery or supercapacitor material,” said Yury Gogotsi of Drexel University, the corresponding author for a forward-looking review paper published in Nature Reviews Materials.
Drexel is a partner university of the Fluid Interface Reactions, Structures, and Transport, or FIRST, center, an Energy Frontier Research Center located at Oak Ridge National Laboratory and funded by the Department of Energy.
For the past 11 years, a group of scientists with the FIRST center focused on electrochemical research has been studying the interfaces of materials for energy storage. “This is the key – this is where action happens in energy storage,” Gogotsi said. “Basically, this is the frontier of energy storage.”
The electronics market is dominated by lithium-ion batteries and supercapacitors. They are used in multiple consumer and industrial applications that require electrochemical energy-storage, or EES, devices, because they are known to operate safely and efficiently in various environments, especially at high or low temperatures.
The electrolyte is an essential component in EES devices. It’s the conducting bridge to transport ions between the positive and negative electrodes. How well this process occurs determines the device’s performance – how quickly the battery can be charged and how much power it can deliver when discharged. Unwanted changes to the electrolyte can also impact the number of charge cycles it can endure before the battery becomes less efficient.
According to the review paper, ionic liquids show promise as a safe alternative to conventional organic electrolytes. Ionic liquids, or ILs, are known to be stable and non-flammable and tend not to evaporate. They can potentially operate up to six volts, which provides the possibility of higher energy density. (A standard household battery is around 1.5 volts, and a lithium-ion battery is 3 to 3.5 volts.)
However, the interaction of ILs with newly developed materials is not well understood. Studies of improved electrodes have recorded faster charge times, but those batteries used conventional electrolytes. ILs tend to charge more slowly; yet, researching advanced electrodes and ILs at the interface could ultimately improve the battery’s or supercapacitor’s performance while taking advantage of the known benefits of ILs.
The team of scientists from ORNL, Drexel, Boston University and University of California, Riverside, suggest a holistic approach so that the entire energy storage device can work successfully.
“The main goal of this forward-looking review is to outline research direction, guide the community where to look for solutions, take advantage of the good things that ionic liquids can offer and solve the existing problems for safer energy storage,” he said.
To push forward with matching thousands of ionic liquids with numerous choices of new advanced battery materials will require computational power, machine learning, and artificial intelligence to handle the massive amounts of data and possible combinations and potential outcomes.
The FIRST EFRC at ORNL employs a computational modeling approach to achieve fundamental understanding and experimentally validated conceptual and computational models of fluid-solid interfaces found in advanced energy systems and devices, including batteries, supercapacitors, and photo- and electrochemical cells.
The center represents a unique approach, bringing together creative, multi-disciplinary scientific teams to tackle the toughest challenges preventing advances in energy technologies.
“Our center’s mission is to achieve fundamental understanding and validated, predictive models of the atomistic origins of electrolyte and coupled electron transport under nanoconfinement. This will enable transformative advances in capacitive electrical energy storage and other energy-relevant interfacial systems,” said ORNL’s Sheng Dai, who leads the FIRST EFRC.
“The deep understanding of electrode material–ionic liquid coupling is part of the equation to accomplish our mission,” he added.
The paper titled, “Electrode material–ionic liquid coupling for electrochemical energy storage,” was co-authored by Xuehang Wang, Babak Anasori and Yury Gogotsi of Drexel University; Maryam Salari, Jennifer Chapman Varela, and Mark W. Grinstaff of Boston University; De-en Jiang of University of California, Riverside; and David J. Wesolowski and Sheng Dai of ORNL.
Reference: “Electrode material–ionic liquid coupling for electrochemical energy storage” by Xuehang Wang, Maryam Salari, De-en Jiang, Jennifer Chapman Varela, Babak Anasori, David J. Wesolowski, Sheng Dai, Mark W. Grinstaff and Yury Gogotsi, 23 July 2020, Nature Reviews Materials.
DOI: 10.1038/s41578-020-0218-9
The research was sponsored by DOE’s Office of Science, though the FIRST EFRC, and by Samsung Electronics Co. (Samsung Advanced Institute of Technology, or SAIT).
Be the first to comment on "Interface of Advanced Materials Key to Safer, Longer-Lasting Energy Storage"