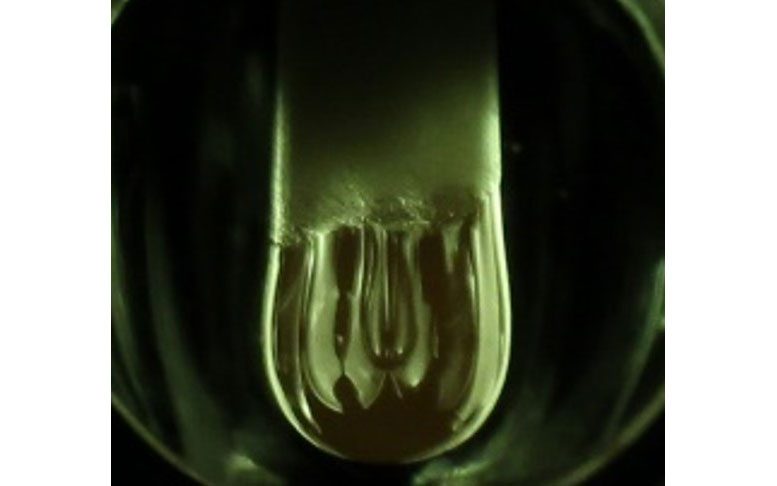
A molten hanging droplet, or pendant (at center), forms from an aluminum oxide rod under intense heat and light from xenon lamps. Using this process, MIT researchers have demonstrated a new electrochemical method to study thermodynamic processes in a molten oxide melt at temperatures above 2,000 degrees Celsius.
The thermodynamic properties of compounds such as aluminum oxide, which are known as refractory materials because they melt at temperatures above 2,000 degrees Celsius (3,632 Fahrenheit), have been difficult to study because few vessels can withstand the heat to contain them, and those that do often react with the melt and contaminate it.
Now MIT researchers are showcasing a container-less electrochemical method to study the thermodynamic properties of these hot melts in a paper published in the Journal of The Electrochemical Society.
“We have a new technique which demonstrates that the rules of electrochemistry are followed for these refractory melts,” says senior author Antoine Allanore, an associate professor of metallurgy. “We have now evidence that these melts are very stable at high temperature, they have high conductivity.”
Adapting a thermal imaging (or arc imaging) furnace more commonly used for floating zone crystal growth, MIT graduate student Brad Nakanishi melted an alumina (aluminum oxide) rod and contacted the liquid pendant droplet that it formed with electrodes, creating an electrochemical cell that allowed decomposition of pure, alumina electrolyte to oxygen gas and aluminum alloy by electrolysis for the first time. The aluminum oxide itself serves as the electrolyte in this electrochemical cell, which operates similarly to water electrolysis.
“Decomposition voltage measurements give us direct access to the quintessential thermodynamic property that is chemical potential, also called Gibbs energy,” Nakanishi explains. “We’ve shown we make electrochemical measurements in a new class of electrolytes, the molten refractory oxides.”
The change in this Gibbs energy, or chemical potential, with respect to temperature is known as entropy. “At high temperatures, entropy is really important and very challenging to predict, so having the ability to measure entropy in these systems is key,” Nakanishi says.
This video of an electrochemical cell shows oxygen bubbles forming as aluminum oxide decomposes into pure aluminum and pure oxygen. In a new paper, MIT researchers demonstrate an electrochemical method to study the thermodynamic properties of these hot liquid alloys by measuring their electrical conductivity properties. Video: Bradley Nakanishi
A hanging droplet
Using this technique, four reflected xenon lamps hone in on the tip of the sample, melting a liquid droplet, which is held to the rod by surface tension and quickly solidifies after the lights are turned off. While the droplet is liquefied, the electrodes are raised into the droplet to complete an electrical circuit, with the liquid alumina itself functioning as the electrolyte.
“That’s something that we have not seen done otherwise, as well, doing electrochemistry in a suspended droplet above 2,000 C,” Nakanishi says.
The hanging droplet has a high surface tension relative to its density.
“The concentration of the light energy, hot zone, and large thermal gradients present, allows us in a very controlled way to create a situation for stable droplet and electrode contact,” Nakanishi says. “It sounds challenging, but the method we’ve refined is straightforward and rapid to operate in practice thanks, in part, to a camera enabling continuous observation of the droplet and electrodes during the experiment.”
Allanore says the stability of the liquid aluminum oxide and a smart choice of electrode materials allow measurement of well-defined energy levels.
“The paper shows that we can now measure fundamental thermodynamic properties of such a melt,” Allanore says. “In the case of molten alumina, we’ve actually been able to study the property of the cathode product. As we decompose aluminum oxide, to oxygen on one side [anode] and aluminum on the other side [cathode], then that liquid aluminum interacts with the electrode, which was iridium in that case,” he says.
Video of the operating cell shows oxygen gas bubbles forming within the cell as the alumina decomposes into aluminum at the cathode (the negatively charged electrode) and pure oxygen at the iridium anode (the positively charged electrode). The aluminum does interact with the iridium cathode, which is confirmed by partial melting and post-experiment images of the microstructure showing an aluminum-iridium alloy deposit.
“We can now calculate the thermodynamic property of that alloy, of that interaction, which is something that was never measured before. It was calculated and predicted. It was never measured. Here in this paper we confirm actually predictions from computation using our method,” Allanore says.
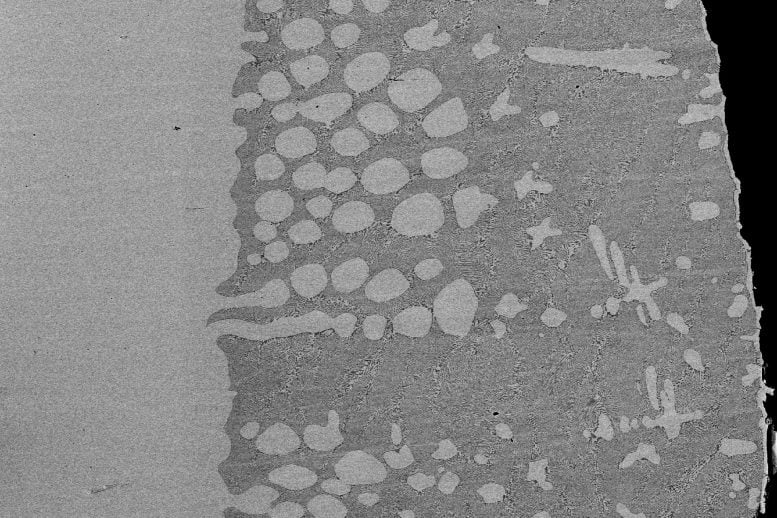
By adapting a thermal imaging furnace more commonly used for growing crystals, MIT graduate student Bradley Nakanishi melted an aluminum oxide rod and contacted the hanging liquid pendant that it formed with electrodes, enabling the decomposition by electrolysis of the material into oxygen gas and aluminum alloy for the first time. Here, a back-scattered electron micrograph shows the variation in composition and microstructure along a vertical cross-section of the cathode after electrolysis. A liquid aluminum-iridium alloy deposit (center-right) forms at the interface between the iridium cathode (left) and electrolyte (black area, far right).
New predictive powers
For key industrial questions, such as how hot a turbine engine can run, engineers need thermodynamic data on both the solid and liquid states of metal alloys, in particular, the transition zone at which a solid melts. “We’re not so great on the liquid state, and at high temperature we also have a lot of trouble measuring Gibbs energy in the liquid state,” Nakanishi says.
“Here we’re adding experimental data,” he says. “We’ve created a method that you can measure the Gibbs free energy of a liquid, so now combined with our ability in a solid, we can start informing things like these transition temperatures among other thermodynamic questions, which are related to material stability.”
The melt is ionic, containing a mix of both negatively charged oxygen anions and neutral oxygen atoms as well as positively charged aluminum cations and neutral aluminum atoms.
“The key significance of Bradley Nakanishi and Antoine Allanore’s research findings is the capability to determine thermodynamic parameters [e.g., thermodynamic activity] at temperatures greater than 1,600 C from the electrochemical measurements for molten oxides, as well as the applicability to a broader electrolyte from a molten oxide to a molten salt,” says University of Texas at El Paso Professor of Mechanical Engineering Arturo Bronson, who was not involved in this research. “In addition, a possible relation of the oxygen partial pressure to the doubly-charged, free oxygen ion will characterize its effect on the associated cations and anions within the molten oxide to explain thermodynamic behavior between the liquid metal and liquid oxide.”
“The quality of the research is a world-class approach developed for difficult experimental studies of ultra-high temperature reactions of liquid metals and liquid oxides, especially with inclusion of electrochemical impedance spectroscopy,” Bronson says. However, a limitation of the study is the uncertainty of the temperature measurements within a range of plus or minus 10 degrees C. “The uncertainty of the measured parameters will ultimately depend on the accuracy of the measured temperature [already at plus or minus 10 kelvins], because the electrochemical parameters [i.e., voltage and current] will clearly depend on the temperature uncertainty,” Bronson explains.
More electrolyte possibilities
Allanore notes that electrochemistry is one of the most selective processing technologies, “but to date it was very challenging to study the electrochemistry with these high temperature melts.”
Electrolyte selection is key for designing new processes for the electrochemical extraction of reactive metals, and the new work demonstrates that more electrolytes are available for extracting metals. “We can now study the solubility of ores containing refractory metal oxides in these melts. So we are basically now adding at least three or four candidate electrolytes that could be used for metal extraction, in particular for what we call reactive metals such as aluminum, niobium, titanium, or the rare earths,” Allanore adds. The research was funded by the U.S. Office of Naval Research.
Future work will focus on applying these high-temperature electrochemical techniques to investigate the potential for selectively separating the rare earth oxides. Though required in only relatively small quantities usually, the individual rare earth elements are essential for high-tech applications, including cell phones and electric vehicles. Well-established methods to concentrate rare earth oxides from their ore produce a mixture of the 14 rare earth oxides, Allanore notes. “If we were using such a rare earth oxide mixture as our electrolyte, we could potentially selectively separate one rare earth metal from the 13 others,” he says.
New, stable materials such as rare earth oxides that can withstand high temperatures are needed for uses as varied as building faster airplanes and extending the lifetime of nuclear power plants. But one country, China, holds a near monopoly over rare earth element production. “The separation of rare earths from each other is the key challenge in making rare earth metals extraction more sustainable and economically feasible,” Nakanishi says.
While the newly published paper examines a single component electrolyte, aluminum oxide by itself, Nakanishi says “our aim is to extend this approach so that we can measure chemical potentials, Gibbs energy, in multi-component electrolytes.”
“This opens up the door to many more candidates for electrolytes that we can use to extract metals, and also make oxygen,” he says.
This ability to exhaust oxygen as a byproduct rather than carbon monoxide or carbon dioxide has potential to reduce greenhouse gas emissions and global warming.
Reference: “Electrochemical Study of a Pendant Molten Alumina Droplet and Its Application for Thermodynamic Property Measurements of Al-Ir” Bradley R. Nakanishi and Antoine Allanore, 25 October 2017, Journal of The Electrochemical Society.
DOI: 10.1149/2.1091713jes
Now MIT researchers are showcasing a container-less electrochemical method to study the thermodynamic properties of these hot melts in a paper published in the Journal of The Electrochemical Society.
https://basketball-legends.co