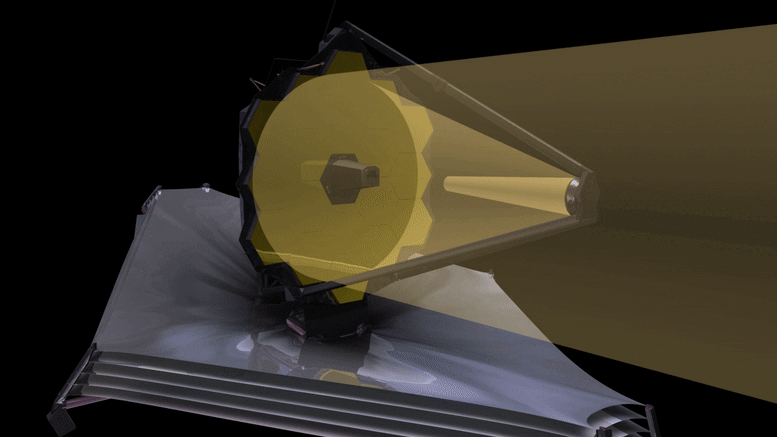
This animation shows the path light will follow as it hits the primary James Webb Space Telescope (JWST) mirror, and is reflected to the secondary, and then in through the aft optics assembly where the tertiary and fine steering mirrors are. The light is then reflected and split and directed to the science instruments by pick-off mirrors. JWST is a three-mirror anastigmat telescope. Credit: NASA, ESA, and G. Bacon (STScI)
One of the James Webb Space Telescope’s four primary scientific instruments, known as NIRISS, has concluded its postlaunch preparations and is now ready for science. NIRISS, which stands for Near-Infrared Imager and Slitless Spectrograph instrument, provides observing modes for slitless spectroscopy, high-contrast interferometric imaging, and imaging, at wavelengths between 0.6 and 5.0 μm over a 2.2′ x 2.2′ FOV. It will be used to investigate the following science objectives: first light detection, exoplanet detection and characterization, and exoplanet transit spectroscopy.
The final NIRISS mode to be checked off before the instrument was certified ready to begin scientific operations was the Single Object Slitless Spectroscopy (SOSS) capability. The heart of the SOSS mode is a specialized prism assembly that disperses the light of a cosmic source to create three distinctive spectra (rainbows), revealing the hues of more than 2,000 infrared colors collected simultaneously in a single observation.
This mode will be specifically used to probe the atmospheres of transiting exoplanets, i.e., planets that happen to eclipse their star periodically, momentarily dimming the star’s brightness for a period of time. By comparing the spectra collected during and before or after a transit event with great precision, it is possible to determine not only whether or not the exoplanet has an atmosphere, but also what atoms and molecules are in it.

The Image Behind the Spectrum. This is a test detector image from the NIRISS instrument operated in its single-object slitless spectroscopy (SOSS) mode while pointing at a bright star. Each color seen in the image corresponds to a specific infrared wavelength between 0.6 and 2.8 microns. The black lines seen on the spectra are the telltale signature of hydrogen atoms present in the star. NIRISS is a contribution from the Canadian Space Agency (CSA) to the Webb project that provides unique observational capabilities that complement its other onboard instruments. Credit: NASA, CSA, and NIRISS team/Loic Albert, University of Montreal
“I’m so excited and thrilled to think that we’ve finally reached the end of this two-decade-long journey of Canada’s contribution to the mission. All four NIRISS modes are not only ready, but the instrument as a whole is performing significantly better than we predicted. I am pinching myself at the thought that we are just days away from the start of science operations, and in particular from NIRISS probing its first exoplanet atmospheres,” said René Doyon, principal investigator for NIRISS, as well as Webb’s Fine Guidance Sensor, at the University of Montreal.
With NIRISS postlaunch commissioning activities concluded, the Webb team will continue to focus on checking off the remaining five modes on its other instruments. NASA’s James Webb Space Telescope, a partnership with ESA (European Space Agency) and CSA, will release its first full-color images and spectroscopic data on July 12, 2022.
Written by:
- Thaddeus Cesari, NASA’s Goddard Space Flight Center
- Nathalie Ouellette, Webb outreach scientist, Université de Montréal
Electromagnetic (EM) emissions, with wavelengths in the range defined as Infrared, do not have a “color.” Color is the subjective experience of the human eye and brain, interpreting the relative proportions of visible light, of different wavelengths, captured by the eye. The human eye does not respond to EM radiation in the infrared region. It is invisible, and therefore cannot have a “color” since it can’t be seen.
Spencer computer can see wavelengths coordinate with results to aid humans in seeing colors. Each paint at paint catalog of colors. Wavelengths & put two to two or 1 to 1 and so on. Been able to preform that task for decades like half a century. Looking forward to more exploring.
Yes, one can map the intensity of pixels for a particular invisible wavelength or range of wavelengths to red, green, and/or blue of the display device. What results is known as a false-color rendition. Again, IR wavelengths have no actual color, as perceived by humans. The false-colors resulting are not constant or even determined by the wavelength of the IR emissions. The perceived colors are determined by which IR wavelengths are sent to which three primary color sources of the display device. And, because the display device only has three primary colors, all 2,000 regions of the IR cannot be displayed simultaneously. One must choose 2 or 3 regions out of the 2,000 available, and decide which primary color to map those 2 or 3 regions to. One can expand the use of the original data by using ratios of 6 ‘bands’ out of the available 2,000. Although, band ratios tend to be very noisy, especially when the denominator is very large. However, fundamentally, the IR ‘bands’ that can be displayed for human perception at any one time are a very small subset of the 2,000 resolved. The false-colors perceived by an observer are not unique to the wavelength(s) but are determined by the mapping strategy and the adjustment of the three histogram adjustments that determine the intensities sent to the display device.
Computers don’t “see” anything. They arithmetically manipulate the intensities of signals that are displayed as pixels on a screen where the position on the screen corresponds to the position measured in the original scene, and the recorded intensity determines either the monochromatic display intensity, or the true-color if the emissions were in the range that can stimulate the rods and cones of the human eye. Otherwise, one is dealing with pseudo-colors or false-colors.
Go CSA and Montreal U! Let’s find those life-possible planets so we have an exact point to transmit r u there messages!
Get ready for even more mysteries to arise due to the ability of this telescope. Nothing will ever makes sense as long as the Lambda-CDM model is used to interpret the findings.
The only way to understand what will be found is to realize that 13.8 billion years ago, the “Big Bang”was simply our universe turning itself into a gargantuan particle collider. Gravity had absolutely nothing to do with the energy we see. Each and every galaxy has cooled ever since this collision happened. That is the way the second law of thermodynamics works. The first law is followed by assuming everyone was already here in a possibly infinite, static universe. Newton’s third law is followed by assuming it is nothing but momentum from the collision that made the galaxies expand. Whatever is found from the telescope must be applied to the laws of physics.
LMAO they are using an iphone flashlight and a prism. In any case, can they turn the thing on already, need to see the damn big bang. F***ing show the beginning of the universe, in multiple spectras of color if you need to, but we need to see everything pop into existence or some s***