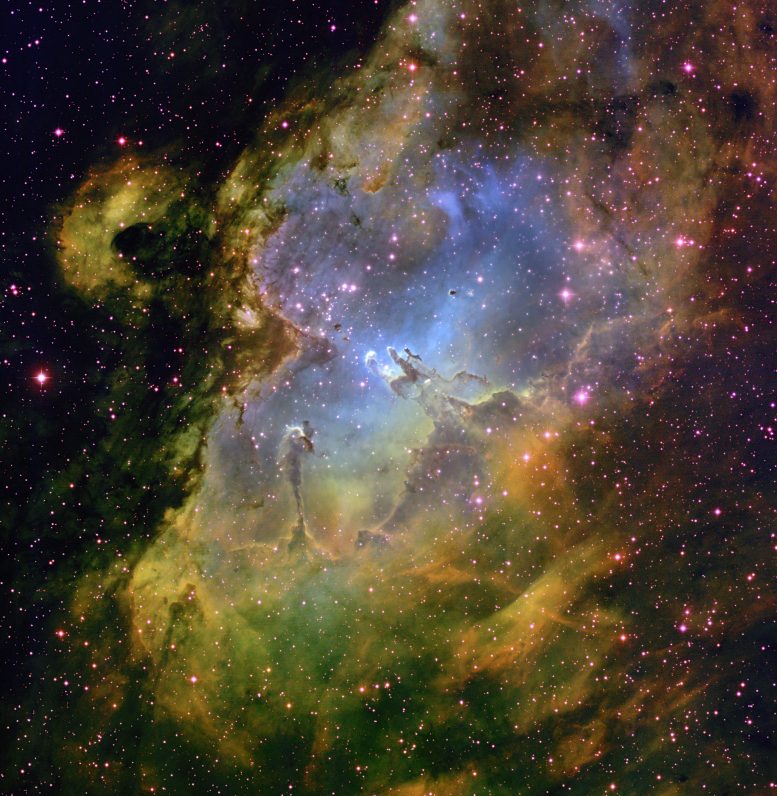
In the Eagle Nebula stars are formed amid the tall pillars and round globules of dark dust and cold molecular gas. Credit: T. A. Rector & B. A. Wolpa, NOAO, AURA
A team of scientists from the University of Leeds has discovered that chemical reactions once thought to be ‘impossible’ in the coldness of space can actually occur thanks to a phenomenon called ‘quantum tunneling.’
New research has revealed that chemical reactions previously thought to be ‘impossible’ in space actually occur ‘with vigor,’ a discovery that could ultimately change our understanding of how alcohols are formed and destroyed in space – and which could also mean that places like Saturn’s moon Titan, once considered too cold for life to form, may have a shortcut for biochemical reactions.
A team at the University of Leeds, UK recreated the cold environment of space in the laboratory and observed a reaction of the alcohol methanol and an oxidizing chemical called the ‘hydroxyl radical’ at minus 210 degrees Celsius. They found that not only do these gases react to create methoxy radicals at such an incredibly cold temperature, but that the rate of reaction is 50 times faster than at room temperature.
They also found that this faster than expected reaction can only occur in the gas phase in space, that a product is formed (CH3O) – and that it can only form via a phenomenon they call ‘quantum tunneling.’
As research leader Dwayne Heard, Professor of Atmospheric Chemistry at the School of Chemistry, University of Leeds explains, quantum tunneling is a ‘non-classical phenomenon,’ which means that the wave function for the interaction of OH with methanol has ‘a non-zero probability of extending beyond the barrier to reaction.’ This means that the system can emerge on the ‘product side’ of the reaction without having to go ‘over the top of the reaction barrier.’
In other words, the tunneling phenomenon is based on the quirky rules of quantum mechanics, which contend that particles do not tend to have defined states, positions, and speeds, but instead exist in a haze of probability. This means that although a given particle might have a strong probability of being on one side of a barrier, there is still a very small chance of it actually being found on the other side of it – in effect allowing it to occasionally ‘tunnel’ through a wall that would otherwise be impenetrable.
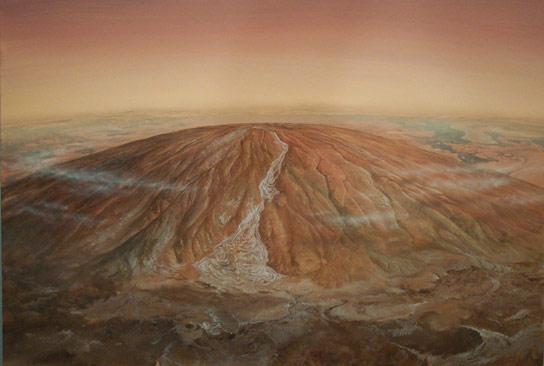
An artist’s impression of Ganesa Macula, a mountain on Saturn’s moon Titan believed to be an “ice volcano” that periodically belches “lava” containing liquid water. This water may react with organic compounds in Titan’s atmosphere to create complex molecules similar to those on the early Earth. Credit: Michael Carroll
“Chemical reactions get slower as temperatures decrease, as there is less energy to get over the ‘reaction barrier.’ But quantum mechanics tells us that it is possible to cheat and dig through this barrier instead of going over it. This is called ‘quantum tunneling,’” says Heard.
Put simply, Heard says that the research shows that organic chemistry can occur in space, here converting an alcohol into an alkoxy radical – which can then go on to form a carbonyl group such as formaldehyde.
“So we are showing that one functional group can be converted to another despite the cold conditions of space. Reactions that were discounted in space because it was too cold may now occur – owing to the tunneling,” he adds.
The research, summarized in the recent Nature Chemistry paper ‘Accelerated chemistry in the reaction between the hydroxyl radical and methanol at interstellar temperatures facilitated by tunneling,’ also demonstrates that such quantum tunneling reactions can occur in a wide range of environments, including cold planetary atmospheres, star-forming regions, stellar outflows, and circumstellar envelopes.
‘Key Interstellar Molecule’
Commenting on the findings, Dr. Robin T. Garrod, Senior Research Associate at the Center for Radiophysics & Space Research, Cornell University, says that methanol (CH3OH) is a ‘key interstellar molecule’ that is ‘crucial to complex organic chemistry in interstellar and star-forming environments.’ “It acts as the feed-stock for various more complex organic molecules during the star-formation process, providing a molecular building-block from which more complex structures may form. Understanding how it is destroyed – and thus whether and how its vestigial molecular structure is passed on to its destruction products – is therefore important to our understanding of the evolution of chemical complexity from interstellar clouds to star and planet formation,” he explains.
Methanol is also interesting to scientists because it appears to have no gas-phase formation mechanism of its own, in spite of its ubiquity in interstellar space. He points out that recent chemical kinetics models rely on its formation from carbon monoxide (CO) on interstellar dust-grain surfaces – where he says it has been ‘detected in abundance’ through Infrared (IR) absorption studies of interstellar clouds. These models assume that a small portion of the surface-formed methanol is sublimated into the gas phase, where it is detected through mm/sub-mm emission spectroscopy to have an abundance around a thousand times lower than on the grains in cold regions of around 10K.
“The very existence of methanol in space underscores the delicate interplay between different chemical phases – gas phase and surface chemistry – in the interstellar medium,” says Garrod.
In Garrod’s view, the University of Leeds work has several implications. Most directly, he says it provides a ‘neat explanation’ for the recent detection of abundant CH3O toward object B1-b (a recently detected low-mass protostar). Moreover, he says the new work shows a ‘strong bias’ toward the production of CH3O over CH2OH at the low temperatures prevalent in interstellar clouds, through the hitherto ‘little-considered’ OH+CH3OH reaction.
“In general, little is known of gas-phase reactions at very low temperature, although it is becoming increasingly clear that quantum effects can be critical to low-temperature reaction rates for many processes that are relevant to interstellar chemistry,” he says.
“Some key low-temperature reactions don’t appear to obey the expectations based on room-temperature behavior. They no longer display the classical Arrhenius-type behavior that one might expect. Low temperatures mean that slow, non-thermal processes (i.e. quantum tunneling) can dominate the reaction process,” he adds.
For Garrod, the new work also indicates that reactive radicals such as CH3O may be important in gas-phase chemistry, as well as dust-grain surface chemistry – to ‘perhaps produce more complex species.’ “Recent detections of molecules such as methyl formate (HCOOCH3) and dimethyl ether (CH3OCH3) in interstellar clouds at very low temperatures – meaning not associated with relatively high temperatures caused by star formation – have still not been adequately explained. Gas-phase reactions such as the one investigated here may play a role in this process,” he says.
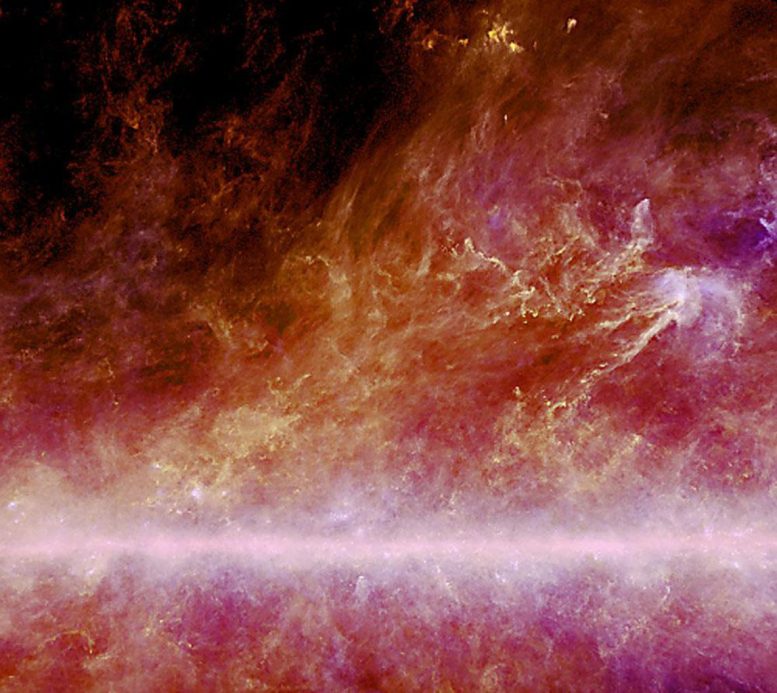
The nearby Milky Way galaxy in cold dust. This remarkable dust tapestry was resolved in detail in a wide region of the sky imaged in far-infrared light — the image is a digital fusion of three infrared colors. Red corresponds to temperatures as cold as 10 degrees Kelvin, while white corresponds to gas as warm at 40 Kelvins. The pink band across the lower part of the image is warm gas confined to the plane of our galaxy. The bright regions typically hold dense molecular clouds that are slowly collapsing to form stars, whereas the dimmer regions are most usually diffuse interstellar gas and dust. Why these regions have intricate filamentary shapes shared on both large and small scales remains a topic of research. Future study of the origin and evolution of dust may help in understanding the recent history of our galaxy as well as how planetary systems such as our solar system came to be born. Credit: ESA, Planck HFI Consortium, IRAS
“This investigation also points to the possibility that other, similar reactions may be affected by the consideration of hydrogen-bonding at low temperatures, allowing a sufficiently stable intermediate to form for quantum tunneling processes to shape the formation of products,” he adds. However, Garrod argues that there is still ‘a great deal of work’ to be done in this field – and stresses that our understanding of low-temperature gas-phase chemistry is ‘far from complete.’ He also highlights the fact that there is potential for a range of other gas-phase processes to be ‘influential in the formation of complex chemical structures in interstellar space and during the star-formation process.’ For him, the work may also have implications for the chemistry that occurs on dust-grain surfaces in the interstellar medium (ISM). Current astronomical observations suggest a tendency toward organics with a C-O-C structure that may result from the ‘preferential’ formation of CH3O radicals over the isomeric CH2OH form, in line with these results – and Garrod points out that an ‘enormous and growing’ number of complex organic molecules appear to be formed on dust grain surfaces at moderate (~30 – 100 K) temperatures during the star-formation process.
“Both OH and CH3OH are likely to be abundant in the ice mantles that form on interstellar dust grains, and in which organic chemistry is expected to occur. The new findings may suggest a similar bias toward CH3O-related chemistry on dust grains as the investigated reaction may produce in the gas phase,” says Garrod.
“However, it is unclear to what degree the reaction kinetics of the gas-phase processes investigated here may be applied to the same or similar reactions taking place on surfaces or within an ice matrix,” he adds.
Although more research is needed, the revelation that such cold chemistry reactions can occur in cold planetary atmospheres, as well as star-forming regions, stellar outflows, and circumstellar envelopes, is likely to excite a good deal of interest in the astrobiology community – and help to boost the chances that such complex reactions occur with frequency in places like Titan.
Reference: “Accelerated chemistry in the reaction between the hydroxyl radical and methanol at interstellar temperatures facilitated by tunnelling” by Robin J. Shannon, Mark A. Blitz, Andrew Goddard and Dwayne E. Heard, 30 June 2013, Nature Chemistry.
DOI: 10.1038/nchem.1692
How about we have a moratorium on the word “that”. If all the unnecessary “thats” were removed from this article it would be several lines shorter…it is that bad…and quite annoying as well. Haven’t these writers taken any English grammar courses?
QM makes my brain hurt
This makes me so happy 🙂
This is outstanding news. Very exciting to think that life could be so much more abundant even in interstellar space!
What was wrong with my comment yesterday?
Nothing, we are currently updating the Spam filters and that was accidentally filtered…it has been pulled out. Thanks for following up.