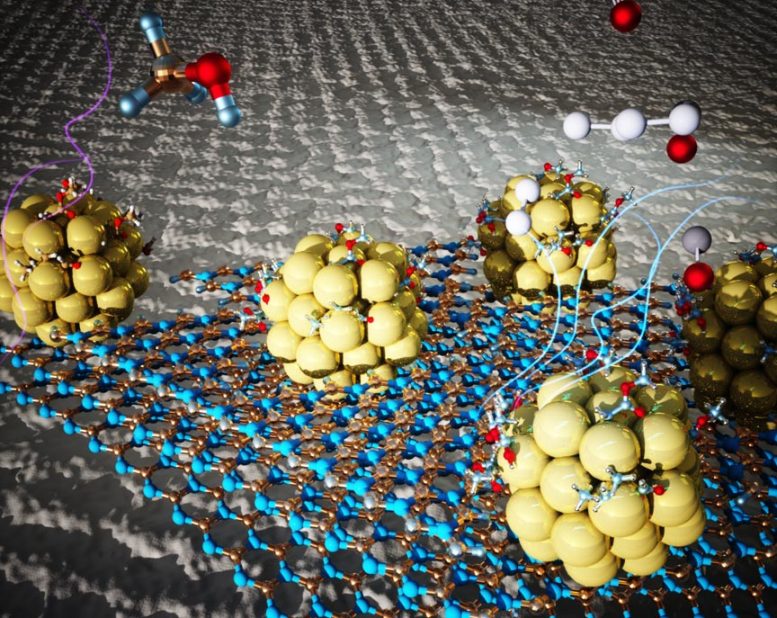
An illustration of the 2D boron nitride substrate with imperfections that host tiny nickel clusters. The catalyst aids the chemical reaction that removes hydrogen from liquid chemical carriers, making it available for use as a fuel. Credit: Jeff Urban/Berkeley Lab
A new nanomaterial helps obtain hydrogen from a liquid energy carrier, in a key step toward a stable and clean fuel source.
Hydrogen is a sustainable source of clean energy that avoids toxic emissions and can add value to multiple sectors of the economy including transportation, power generation, and metals manufacturing, among others. Technologies for storing and transporting hydrogen bridge the gap between sustainable energy production and fuel use, and therefore are an essential component of a viable hydrogen economy.
But traditional means of storage and transportation are expensive and susceptible to contamination. As a result, researchers are searching for alternative techniques that are reliable, low-cost, and simple. More efficient hydrogen delivery systems would benefit many applications such as stationary power, portable power, and mobile vehicle industries.
Now, as reported in the journal Proceedings of the National Academy of Sciences, researchers have designed and synthesized an effective material for speeding up one of the limiting steps in extracting hydrogen from alcohols. The material, a catalyst, is made from tiny clusters of nickel metal anchored on a 2D substrate.
The team led by researchers at Lawrence Berkeley National Laboratory’s (Berkeley Lab) Molecular Foundry found that the catalyst could cleanly and efficiently accelerate the reaction that removes hydrogen atoms from a liquid chemical carrier. The material is robust and made from earth-abundant metals rather than existing options made from precious metals, and will help make hydrogen a viable energy source for a wide range of applications.
“We present here not merely a catalyst with higher activity than other nickel catalysts that we tested, for an important renewable energy fuel, but also a broader strategy toward using affordable metals in a broad range of reactions,” said Jeff Urban, the Inorganic Nanostructures Facility director at the Molecular Foundry who led the work.
The research is part of the Hydrogen Materials Advanced Research Consortium (HyMARC), a consortium funded by the U.S. Department of Energy’s Office of Energy Efficiency and Renewable Energy Hydrogen and Fuel Cell Technologies Office (EERE). Through this effort, five national laboratories work towards the goal to address the scientific gaps blocking the advancement of solid hydrogen storage materials. Outputs from this work will directly feed into EERE’s H2@Scale vision for affordable hydrogen production, storage, distribution, and utilization across multiple sectors of the economy.
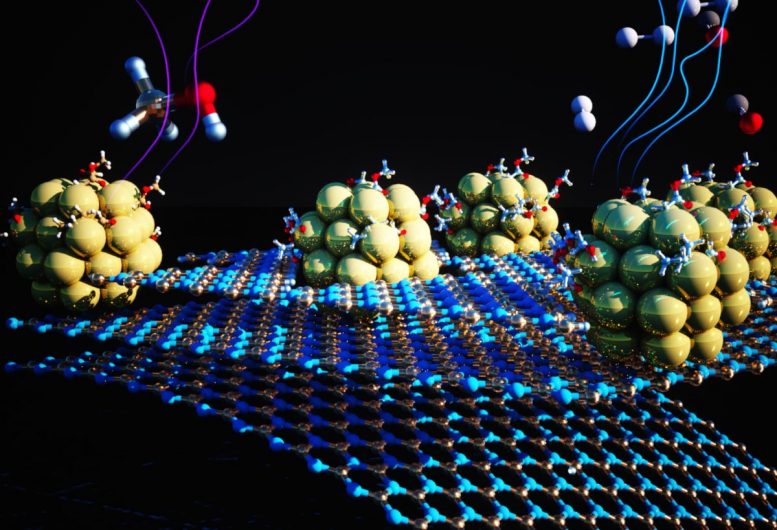
Illustration of the 2D boron nitride substrate with imperfections that host tiny nickel clusters. The catalyst aids the chemical reaction that removes hydrogen from liquid chemical carriers, making it available for use as a fuel. Credit: Jeff Urban/Berkeley Lab
Chemical compounds that act as catalysts like the one developed by Urban and his team are commonly used to increase the rate of a chemical reaction without the compound itself being consumed—they might hold a particular molecule in a stable position, or serve as an intermediary that allows an important step to be reliably to completed. For the chemical reaction that produces hydrogen from liquid carriers, the most effective catalysts are made from precious metals. However, those catalysts are associated with high costs and low abundance, and are susceptible to contamination. Other less expensive catalysts, made from more common metals, tend to be less effective and less stable, which limits their activity and their practical deployment into hydrogen production industries.
To improve the performance and stability of these earth-abundant metal-based catalysts, Urban and his colleagues modified a strategy that focuses on tiny, uniform clusters of nickel metal. Tiny clusters are important because they maximize the exposure of reactive surfaces in a given amount of material. But they also tend to clump together, which inhibits their reactivity.
Postdoctoral research assistant Zhuolei Zhang and project scientist Ji Su, both at the Molecular Foundry and co-lead authors on the paper, designed and performed an experiment that combatted clumping by depositing 1.5-nanometer-diameter nickel clusters onto a 2D substrate made of boron and nitrogen engineered to host a grid of atomic-scale dimples. The nickel clusters became evenly dispersed and securely anchored in the dimples. Not only did this design prevent clumping, but its thermal and chemical properties greatly improved the catalyst’s overall performance by directly interacting with the nickel clusters.
“The role of the underlying surface during the cluster formation and deposition stage has been found to be critical, and may provide clues to understanding their role in other processes,” said Urban.
Detailed X-ray and spectroscopy measurements, combined with theoretical calculations, revealed much about the underlying surfaces and their role in catalysis. Using tools at the Advanced Light Source, a DOE user facility at Berkeley Lab, and computational modeling methods, the researchers identified changes in the physical and chemical properties of the 2D sheets while tiny nickel clusters occupy pristine regions of the sheets and interact with nearby edges, thus preserving the tiny size of the clusters. The tiny, stable clusters facilitated the action in the processes through which hydrogen is separated from its liquid carrier, endowing the catalyst with excellent selectivity, productivity, and stable performance.
Calculations showed that the catalyst’s size was the reason its activity was among the best relative to others that have recently been reported. David Prendergast, director of the Theory of Nanostructured Materials Facility at the Molecular Foundry, along with postdoctoral research assistant and co-lead author Ana Sanz-Matias, used models and computational methods to uncover the unique geometric and electronic structure of the tiny metal clusters. Bare metal atoms, abundant in these tiny clusters, more readily attracted the liquid carrier than did larger metal particles. These exposed atoms also eased the steps of the chemical reaction that strips hydrogen from the carrier, while preventing the formation of contaminants that may clog the surface of the cluster. Hence, the material remained free of pollution during key steps in the hydrogen production reaction. These catalytic and anti-contamination properties emerged from the imperfections that had been deliberately introduced to the 2D sheets and ultimately helped keep the cluster size small.
“Contamination can render possible non-precious metal catalysts unviable. Our platform here opens a new door to engineering those systems,” said Urban.
In their catalyst, the researchers achieved the goal of creating a relatively inexpensive, readily available, and stable material that helps to strip hydrogen from liquid carriers for use as a fuel. This work came out of a DOE effort to develop hydrogen storage materials to meet the targets of EERE’s Hydrogen and Fuel Cell Technologies Office and to optimize the materials for future use in vehicles.
Future work by the Berkeley Lab team will further hone the strategy of modifying 2D substrates in ways that support tiny metal clusters, to develop even more efficient catalysts. The technique could help to optimize the process of extracting hydrogen from liquid chemical carriers.
Reference: “Enhanced and stabilized hydrogen production from methanol by ultrasmall Ni nanoclusters immobilized on defect-rich h-BN nanosheets” by Zhuolei Zhang, Ji Su, Ana Sanz Matias, Madeleine Gordon, Yi-Sheng Liu, Jinghua Guo, Chengyu Song, Chaochao Dun, David Prendergast, Gabor A. Somorjai and Jeffrey J. Urban, 24 November 2020, Proceedings of the National Academy of Sciences.
DOI: 10.1073/pnas.2015897117
The Molecular Foundry and the Advanced Light Source are DOE Office of Science user facilities at Berkeley Lab.
The research was supported by the DOE Office of Science and EERE’s Hydrogen and Fuel Cell Technologies Office.
It would have been helpful if a simple cost-benefit and efficiency comparison was spelled out conservatively.
Why cannot hydrogen substrates be fitted into vehicle tanks and the hydrogen produced be tanked off seperately instead of storage like fuel stations
QUOTE:
“Hydrogen is a sustainable source of clean energy that avoids toxic emissions”
That is INCORRECT when talking about life on the surface of the Earth:
Hydrogen is NOT found here on Earth in a free-form (H2 – molecular Hydrogen); it must be released from chemical bonds to other atoms/molecules (for example H20 – water), and the energy that it takes to break those bonds is the energy that is (partially) stored in the resulting Hydrogen. Then, when the Hydrogen releases that stored energy to do work (make a motor run), that latter process is “clean”.
Therefore, Hydrogen is not a “source” of energy for us to use. It is more like a “battery” for us; not a “fuel” like “gas” (petrol-gasoline, oil, propane, methane-NG, etc.). ((A space-ship traveling through interstellar space may “collect” the free-Hydrogen out there to use as a “source” of energy to fuel its rockets.)) Furthermore, if the energy used to break the Hydrogen free (split the water molecule, etc.) from the chemical bonds that we find it in is not itself “clean”, we can not consider the use of said Hydrogen to be “clean energy”.
When most people think of Hydrogen as a “fuel” for a vehicle (for example), they think of it as a singular source of energy that will be used to power a motor; and now-a-days the concept is typically only presented in terms of a reverse-electrolizer that makes electricity from the Hydrogen that is then used to power an electric motor.
They don’t realize that Hydrogen is the perfect “booster” fuel that opens the door to most other fuels that are too poor in quality to “burn” efficiently and/or cleanly. By simply adding Hydrogen to any fuel at a rate of about 5% of the BTUs, that fuel will burn completely and 100% “clean” in terms of the particulate emissions that send thousands of kids to the ER every year (in the U.S. alone), where many still die, from acute asthma attacks (see the American Lung Association website). In other words, California need not be choked under a blanket of smog, and “we” have known this since at least the 1960s! They already use this tech for diesel-powered fork lifts in giant warehouses (since the 1980s? I think). While it has been hard to store large enough quantities of Hydrogen on a vehicle to use as a singular source of energy (until carbon-fiber tanks became good enough to rate at 10,000psi) and still get a decent travel range (just like BEVs), we could have – and still can – produce vehicles that mix Hydrogen into existing fuel systems and not only eliminate particulate emissions, but get better gas mileage; AND we could eliminate the catalytic converter (that limits power and mileage) in gasoline vehicles, and DEF systems in diesels, both expensive-tech add-ons. Meanwhile, your motor will run COOLER and last longer.
But Hydrogen opens the door to using ANY fuel in your spark-ignition motor, given the correct fuel injectors; not only gasoline, but diesel, veggie oil, cheap wine (alcohol with a high percentage of water), vapors from rotting compost piles, etc.
Electric vehicles rely on batteries that take a global industrial consortium of mining, production, and distribution. Gasoline and diesel rely on a similar global industrial consortium. These consortiums are controlled by a few of the world’s rich and elite. Hydrogen can be made at home or even at the local “quickie-mart”. The other fuels that it can “HyBoost” can be made at home.
Those that control the world do so with banks more than guns. The U.S. dollar was chosen to be the currency of all petro-oil sold in the world in all countries, so that control of the world can be obtained. They fear hydrogen because it undermines the system that they have created.
Actually, hydrogen is likely to be an energy source. Google up “Proton Technologies” and pick your article about them. Still in the R&D stage, but they believe they can break up hydrocarbons down-hole, so that only hydrogen leaves the ground – for 70 cents per kg, which is a quarter of the cost of electrolysed hydrogen.
The oil industry may just morph into a hydrogen-extraction industry.
The “source” is still hydrocarbons. The company’s page:
https://proton.energy/proton-process/
is vague as to how much energy must be introduced into the system to release the hydrogen which is an energy carrier; quotes:
“Oxygen-enhanced air is produced at the wellhead, and then injected deep into the reservoir…”
“Targeted portions of the reservoir become very warm. Where necessary, the temperatures are heightened further through radio frequency emissions.”
“A small part of the energy extracted from the reservoir – as hydrogen, heat or syngas – may be used directly at the wellhead to produce the oxygen-enhanced air, and to operate the pumps.”
So we gotta (a) make oxygen-enhanced air, (b) pump it deep underground under pressure (where it oxidates heavy hydrocarbons and creates heat to split other hydrocarbons and water), and (c) further heat up the deep ground with RF energy. All that takes an input of energy to start with; and they don’t say how much RF energy is needed. But it LOOKS promising.
If it produces a net gain of usable energy in the end, which is what this company seems to suggest, then one might say that yes, that the hydrogen produced is a SOURCE of energy for us. It’s not often you get sumptin’ fo’ notin’, though.
And where do we get the oxygen from? The air we breathe? Then the atmosphere still gets a higher overall percentage of CO2. And then we pump that oxygen underground where it stays until when? How long can we do that for, especially as the global economy is growing exponentially, before the air gets too thin to hike up a 10,000 foot mountain – 50 years? 100 years? Not trying to bash the new tech, just asking questions…
If we split ocean water with solar/wind derived energy, we get hydrogen and oxygen. When the hydrogen is consumed so the solar/wind energy stored in it does work, the exhaust is absolute pure water (bonus: drinking water in the desert, or other locations where clean water and filters are sparse!), and the loop is closed: no net loss or gain of oxygen, water, or pollutants, etc…
My interest still lies with tuning a pulsed DC current (or maybe somehow an AC current) to the resonant frequency of water molecules; then they vibrate apart like a crystal wine glass shatters when the lady sings the high-note. Much less energy input (higher efficiency) to split the water. Or so goes the theory…
It doesn’t matter if the source of the hydrogen is hydrocarbons, if the carbon part never leaves the ground.
In the end, for any energy-extraction industry, it only matters if they get more energy out than goes into the extraction effort; and that the energy input does not HAVE to release carbon.
Right now, every wind turbine is made from steel smelted with coal; in a foundation of concrete baked with natural gas; and dug by a backhoe burning diesel. But all of those things can be done with clean energy, and soon enough will be. So it is with other energy-extraction industries like biomass and solar.
If the Proton bunch can poke holes all over the Earth and bring up nothing but hydrogen, with processes that eventually are all-green themselves, then it’s a complete climate win. That’s all that counts.
… Waiting for Godot …