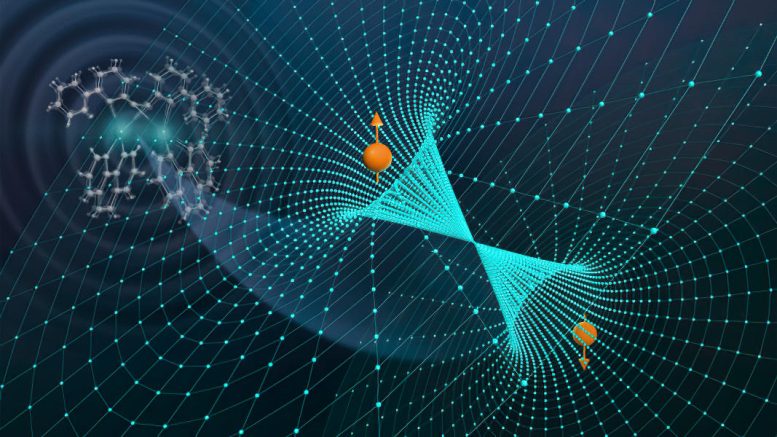
A molecule with two platinum atoms absorbs a photon and starts to vibrate. The vibration enables the molecule’s electron spin to flip, allowing the system to simultaneously change electronic states in a phenomenon called inter-system crossing. Credit: Argonne National Laboratory
Ultrafast lasers and X-rays have revealed the coupling between electronic and nuclear dynamics in molecules.
Nearly a century ago, physicists Max Born and J. Robert Oppenheimer developed a hypothesis about the functioning of quantum mechanics within molecules. These molecules consist of complex systems of nuclei and electrons. The Born-Oppenheimer approximation postulates that the movements of nuclei and electrons within a molecule occur independently and can treated separately.
This model works the vast majority of the time, but scientists are testing its limits. Recently, a team of scientists demonstrated the breakdown of this assumption on very fast time scales, revealing a close relationship between the dynamics of nuclei and electrons. The discovery could influence the design of molecules useful for solar energy conversion, energy production, quantum information science, and more.
The team, including scientists from the U.S. Department of Energy’s (DOE) Argonne National Laboratory, Northwestern University, North Carolina State University, and the University of Washington, recently published their discovery in two related papers in Nature and Angewandte Chemie International Edition.
“Our work reveals the interplay between the dynamics of electron spin and the vibrational dynamics of the nuclei in molecules on superfast time scales,” said Shahnawaz Rafiq, a research associate at Northwestern University and first author on the Nature paper. “These properties can’t be treated independently — they mix together and affect electronic dynamics in complex ways.”
A phenomenon called the spin-vibronic effect occurs when changes in the motion of the nuclei within a molecule affect the motion of its electrons. When nuclei vibrate within a molecule — either due to their intrinsic energy or due to external stimuli, such as light — these vibrations can affect the motion of their electrons, which can in turn change the molecule’s spin, a quantum mechanical property related to magnetism.
In a process called inter-system crossing, an excited molecule or atom changes its electronic state by flipping its electron spin orientation. Inter-system crossing plays an important role in many chemical processes, including those in photovoltaic devices, photocatalysis, and even bioluminescent animals. For this crossing to be possible, it requires specific conditions and energy differences between the electronic states involved.
Since the 1960s, scientists have theorized that the spin-vibronic effect could play a role in inter-system crossing, but direct observation of the phenomenon has proven challenging, as it involves the measurement of changes in electronic, vibrational, and spin states on very fast time scales.
“We used ultrashort laser pulses — down to seven femtoseconds, or seven-millionths of a billionth of a second — to track the motion of nuclei and electrons in real time, which showed how the spin-vibronic effect can drive inter-system crossing,” said Lin Chen, an Argonne Distinguished Fellow, professor of chemistry at Northwestern University and co-corresponding author on both studies. “Understanding the interplay between the spin-vibronic effect and inter-system crossing could potentially lead to new ways to control and exploit the electronic and spin properties of molecules.”
The team studied four unique molecular systems designed by Felix Castellano, a professor at North Carolina State University and co-corresponding author on both studies. Each of the systems is like the other, but they contain controlled, known differences in their structures. This allowed the team to access slightly different inter-system crossing effects and vibrational dynamics to get a fuller picture of the relationship.
“The geometrical changes that we designed into these systems caused the crossing points between the interacting electronic excited states to occur at slightly different energies and under different conditions,” said Castellano. “This provides insight for tuning and designing materials to enhance this crossing.”
Induced by vibrational motion, the spin-vibronic effect in the molecules altered the energy landscape within the molecules, increasing the probability and rate of inter-system crossing. The team also discovered key intermediate electronic states that were integral to the operation of the spin-vibronic effect.
The results were predicted and bolstered by quantum dynamics calculations by Xiaosong Li, a professor of chemistry at the University of Washington and laboratory fellow at DOE’s Pacific Northwest National Laboratory. “These experiments showed very clear, very beautiful chemistry in real time that aligns with our predictions,” said Li, who was an author on the study published in Angewandte Chemie International Edition.
The profound insights unraveled by the experiments represent a step forward in the design of molecules that can make use of this powerful quantum mechanical relationship. This could prove especially useful for solar cells, better electronic displays, and even medical treatments that rely on light-matter interactions.
References:
“Spin–vibronic coherence drives singlet–triplet conversion” by Shahnawaz Rafiq, Nicholas P. Weingartz, Sarah Kromer, Felix N. Castellano and Lin X. Chen, 19 July 2023, Nature.
DOI: 10.1038/s41586-023-06233-y
“Revealing Excited-State Trajectories on Potential Energy Surfaces with Atomic Resolution in Real Time” by Denis Leshchev, Andrew J. S. Valentine, Pyosang Kim, Alexis W. Mills, Subhangi Roy, Arnab Chakraborty, Elisa Biasin, Kristoffer Haldrup, Darren J. Hsu, Matthew S. Kirschner, Dolev Rimmerman, Matthieu Chollet, J. Michael Glownia, Tim B. van Driel, Felix N. Castellano, Xiaosong Li and Lin X. Chen, 28 April 2023, Angewandte Chemie International Edition.
DOI: 10.1002/anie.202304615
Both studies were supported by DOE’s Office of Science. The Nature study was supported in part by the National Science Foundation. Experiments in the Angewandte Chemie International Edition were conducted at the Linac Coherent Light Source at DOE’s SLAC National Accelerator Laboratory. Other authors on the Nature study include Nicholas P. Weingartz and Sarah Kromer. Other authors on the paper published in Angewandte Chemie International Edition include Denis Leshchev, Andrew J. S. Valentine, Pyosang Kim, Alexis W. Mills, Subhangi Roy, Arnab Chakraborty, Elisa Biasin, Kristoffer Haldrup, Darren J. Hsu, Matthew S. Kirschner, Dolev Rimmerman, Matthieu Chollet, J. Michael Glownia and Tim B. van Driel.
you work reveals the interplay between the dynamics of electron spin and the vibrational dynamics of the nuclei in molecules on superfast time scales. These properties can’t be treated independently — they mix together and affect electronic dynamics in complex ways. Congratulations to you all.
So far, the most successful theories describing spacetime are quantum mechanics and relativity. Whether from a quantum perspective or according to Einstein’s theory of relativity, the smallest unit of time is the synchronization of two events. There is no minimum unit of time, only a smaller one. The 2023 Nobel Prize in Physics does not have any cross generational significance. There will be more astonishing achievements in the study of the smallest unit of time. Synchronous effects are common in quantum mechanics. The Nobel Prize in Physics is no different from some so-called academic journals. They firmly believe in:
1. Even without understanding how θ & τ were formed, it can be concluded that they are CP violations.
2. Even if two objects (such as cobalt-60) that rotate in opposite directions are asymmetrical, they are still two objects that mirror each other.
I hope your team has not been fooled by these pseudo scientific ideas.
Great minds at work in a very creative way, keep the creativity alive in science.
@bao
What in the holy flying f*** are you talking about? You’re throwing big words around to sound smart while coming across like a complete buffoon.
Dear Sir or Madam,
Congratulations. You are most qualified to be the best spokesperson for the so-called academic community this year. I sincerely hope that your condemnation statement can be heard by every person who loves science around the world.
He single-handedly murdered the comment section on this science news site. ~