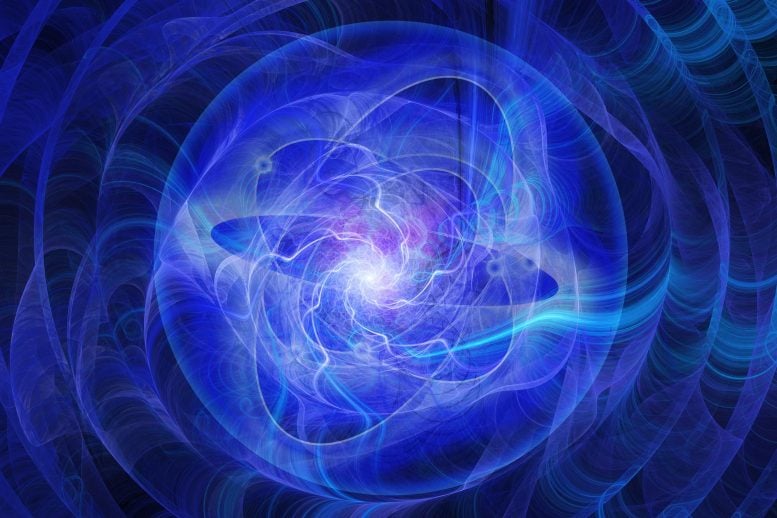
A University of Massachusetts Lowell-led team of nuclear physicists has discovered the symmetry that exists within the core of atoms is not as fundamental as scientists have believed.
A discovery by a team of researchers led by University of Massachusetts Lowell nuclear physicists could change how atoms are understood by scientists and help explain extreme phenomena in outer space.
The breakthrough by the researchers revealed that a symmetry that exists within the core of atoms is not as fundamental as scientists have believed. The discovery sheds light on the forces at work within the nucleus of atoms, opening the door to a greater understanding of the universe. The findings have been published in Nature, one of the world’s premier scientific journals.
The discovery was made when the UMass Lowell-led team was working to determine how atomic nuclei are created in X-ray bursts – explosions that happen on the surface of neutron stars, which are the remnants of massive stars at the end of their life.
“We are studying what happens inside the nuclei of these atoms to better understand these cosmic phenomena and, ultimately, to answer one of the biggest questions in science, how the chemical elements are created in the universe,” said Andrew Rogers, UMass Lowell assistant professor of physics, who heads the research team.
The research is supported by a $1.2 million grant from the U.S. Department of Energy to UMass Lowell and was conducted at the National Superconducting Cyclotron Laboratory (NSCL) at Michigan State University. At the lab, scientists create exotic atomic nuclei to measure their properties in order to understand their role as the building blocks of matter, the cosmos, and of life itself.
Atoms are some of the smallest units of matter. Each atom includes electrons orbiting around a tiny nucleus deep within its core, which contains almost all its mass and energy. Atomic nuclei are composed of two nearly identical particles: charged protons and uncharged neutrons. The number of protons in a nucleus determines which element the atom belongs to on the periodic table and thus its chemistry. Isotopes of an element have the same number of protons but a different number of neutrons.
At the NSCL, nuclei were accelerated to near the speed of light and smashed apart into fragments creating strontium-73 – a rare isotope that is not found naturally on Earth but can exist for short periods of time during violent thermonuclear X-ray bursts on the surface of neutron stars. This isotope of strontium contains 38 protons and 35 neutrons and only lives for a fraction of a second.
Working around the clock over eight days, the team created more than 400 strontium-73 nuclei and compared them to the known properties of bromine-73, an isotope that contains 35 protons and 38 neutrons. With interchanged number of protons and neutrons, bromine-73 nuclei are considered “mirror partners” to strontium-73 nuclei. Mirror symmetry in nuclei exists because of the similarities between protons and neutrons and underlies scientists’ understanding of nuclear physics.
Roughly every half-hour, the researchers created one strontium-73 nucleus, transported it through the NSCL’s isotope separator and then brought the nucleus to a stop at the center of a complex detector array where they could observe its behavior. By studying the radioactive decay of these nuclei, the scientists found that strontium-73 behaved entirely differently from bromine-73. The discovery raises new questions about nuclear forces, according to Rogers.
“Strontium-73 and bromine-73 should appear identical in structure, but surprisingly do not, we found. Probing symmetries that exist in nature is a very powerful tool for physicists. When symmetries break down, that tells us something’s wrong in our understanding, and we need to take a closer look,” Rogers said.
What the scientists saw will challenge nuclear theory, according to Daniel Hoff, a UMass Lowell research associate who was the lead author of the article published in Nature.
“Comparing strontium-73 and bromine-73 nuclei was like looking in a mirror and not recognizing yourself. Once we convinced ourselves that what we were seeing was real, we were very excited,” Hoff said.
Along with Rogers, a resident of Somerville, and Hoff, who lives in Medford, the UMass Lowell team included Physics Department faculty Assistant Prof. Peter Bender, Prof. Emeritus C.J. Lister and former UMass Lowell research associate Chris Morse. Physics graduate students Emery Doucet of Mason, N.H., and Sanjanee Waniganeththi of Lowell also contributed to the project.
As part of the team’s study, state-of-the-art theoretical calculations were carried out by Simin Wang, a research associate at Michigan State, and directed by Witold Nazarewicz, MSU’s John A. Hannah Distinguished Professor of Physics and chief scientist at the Facility for Rare Isotope Beams (FRIB), which will open next year.
The researchers’ work “offers unique insights into the structure of rare isotopes,” Nazarewicz said. “But much still remains to be done. New facilities coming online, such as FRIB at MSU, will provide missing clues into a deeper understanding of the mirror symmetry puzzle. I am glad that the exotic beams delivered by our facility, unique instrumentation and theoretical calculations could contribute to this magnificent work.”
Plans for more experiments are already underway, as the researchers seek to refine and confirm their observations and study these isotopes further.
Reference: “Mirror-symmetry violation in bound nuclear ground states” by D. E. M. Hoff, A. M. Rogers, S. M. Wang, P. C. Bender, K. Brandenburg, K. Childers, J. A. Clark, A. C. Dombos, E. R. Doucet, S. Jin, R. Lewis, S. N. Liddick, C. J. Lister, Z. Meisel, C. Morse, W. Nazarewicz, H. Schatz, K. Schmidt, D. Soltesz, S. K. Subedi and S. Waniganeththi, 1 April 2020, Nature.
DOI: 10.1038/s41586-020-2123-1
I’m not terribly surprised. In the standard model of particles the force sectors are enablers of the charge (C) and “mirror” parity (P) of matter particles. So the CP symmetry breaking that made matter (the matter/antimatter symmetry breaking) must be dispensed out between the neutrino (lepton) and nucleon (quark) sector that makes the tripartite model. (With forces such as Higgs, gluons and electroweak, as well as matter of leptons and quarks that both have their own force interaction matrices with CP breaking possibilities.)
As it happens, preliminary results imply the neutrinos do most of the deed and nucleon constituents almost nothing. But “almost nothing” does not mean absolutely nothing, so small CP breakings such as this mirror-symmetry breaking is seen. The larger question is why neutrinos would take the required part and the quarks just random left overs, which small breakings particle physicists currently describe as “not as fundamental” symmetries.
[In a speculation that may be explained by inflation, since it would give us the simplest possible – most likely – physics which still is complex enough to give us atoms. That may explain the dichotomy in CP breakings as well as why we have only 3 generations (“flavors”) of matter particles and so only one type of Higgs (instead of 4 or more of both). Similarly, neutrinos being without charge puts almost everything of CP breaking into P breaking, with random residuals in the quark sector. The inflation result or our specific universe is finetuned, but only as much as needed as seen in the vacuum energy. Dark energy density could easily been 1,000 times higher or 10 times lower and the universe could still have habitable planets.]
Stupid question: Why would symmetry predict that protons and neutrons would decay identically?
You tell’em, Rusty.
By the time you’re able to observe the structure of the nucleus , atom destabilization has already begun, especially if it is true that Strontium-73 half-life is a fraction of a second ( assuming Bromine-73 may not be). This may give the impression the two are mirrored differently, but in reality, our observational senses based on the tools we have, just may be too slow to capture the moment they are the same. Interesting find, nonetheless, of the half-life of Strontium-73 nuclei. A lot could be explained knowing this, alone.
This is because our standard model of particle physics is wrong. Correct model is given at last chapter of https://vixra.org/abs/1912.0171
… told Ya…
… Gravity is bit odd, I even sometimes ask my self is it a force like others. Is it just an example of some thing that have the same outcome, but the path to a outcome matters too…