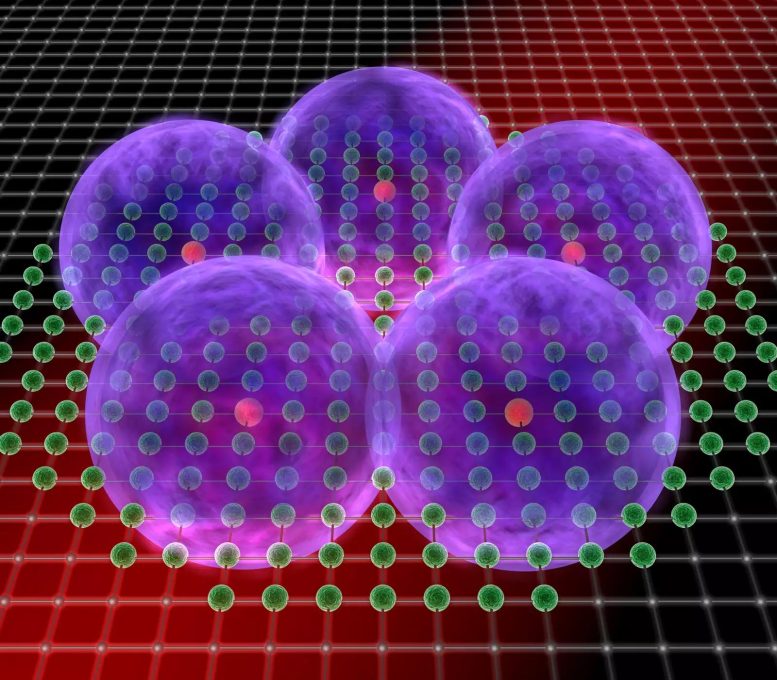
A pentagon of super atoms: The illustration depicts the densest possible ordering of five Rydberg excitations in an ensemble of rubidium atoms that are pinned in an optical lattice. Credit: MPI of Quantum Optics
Scientists investigating how atoms interact with one another have now gained new insights into a type of interaction that may contribute to the advancement of quantum information processing.
Future computers are expected to use the laws of quantum physics to accomplish certain tasks in the blink of an eye that require decades for present-day computers. Physicists at the Max Planck Institute of Quantum Optics in Garching near Munich, have now gained fundamental insights into a particular kind of atomic ensemble – a so-called Rydberg gas – that might play a role in the future design of a quantum computer. They observed how “super atoms” formed in the gas and ordered themselves in geometric shapes such as triangles and squares. In the future, the researchers intend to control the number and geometric configuration of these super atoms. That would be an important step towards a scalable system for quantum information processing.
How and when quantum computers will start operating still remains uncertain. Even so, there are various suggestions for systems they could use to compute with. One example is an ultra-cold ensemble of numerous atoms that are pinned by light in an artificial crystal. The atoms of such a quantum gas should work in concert, similar to the complex way the elements of an electronic circuit solve tricky problems. Scientists working around Immanuel Bloch, Director of the Max Planck Institute of Quantum Optics, are investigating how atoms interact with one another, and they have now gained new insights into a type of interaction that may contribute to the advancement of quantum information processing.
Peter Schauß and his colleagues cooled a gas of several hundred rubidium atoms to a temperature near absolute zero and subsequently loaded them into a so-called optical lattice. Such a lattice is formed by the interference pattern at the intersection of several laser beams, with the light intensity alternatively varying between highs and lows. When the rubidium atoms enter the lattice, they interact with the laser light and migrate to the dimples of what looks like an egg crate. In the end, the atoms turn out to be uniformly distributed over the lattice, with exactly one atom sitting in each dimple.
‘Super atoms’ make it possible to switch states over longer distances.
The scientists subsequently created Rydberg excitations, as they are known, in the atomic ensemble by shining another laser beam onto the gas. Physicists call Rydberg atom a highly excited atom in which one the electrons orbits the nucleus at a much larger distance than it usually does. The size of the atom, that is the region occupied by the electron shell, can then be expanded by more than a thousand times and attain a diameter of several hundred nanometers. Such enormous atoms react very sensitively to electric fields. This year’s Nobel laureate, Serge Haroche, for example, used Rydberg atoms to measure the quantum mechanical state of light particles without destroying them. The extreme sensitivity of Rydberg atoms to electric fields is precisely why they could prove useful for quantum information processing.
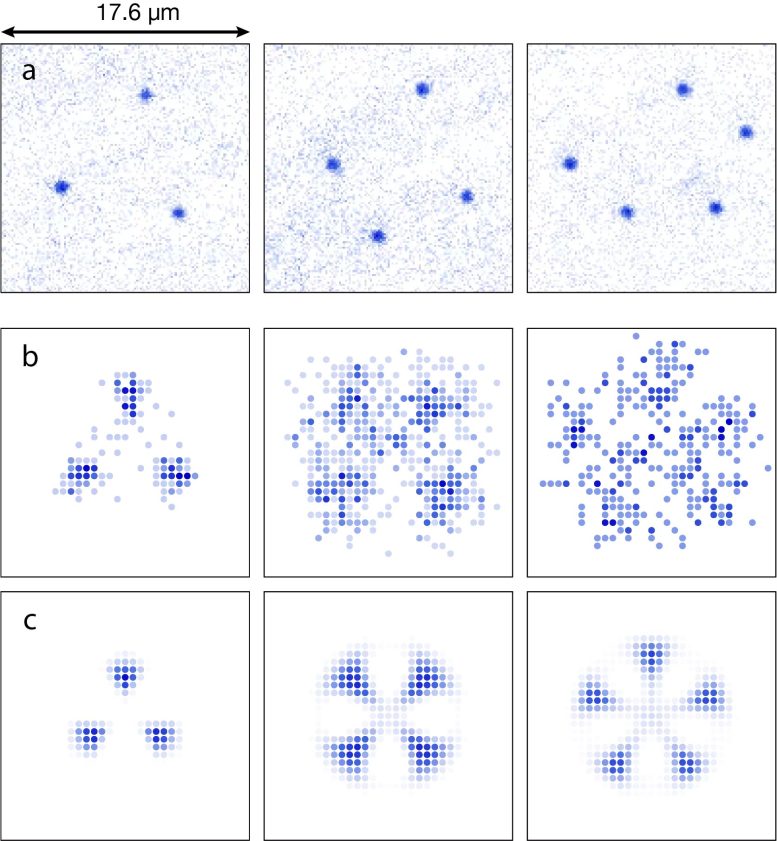
Sorting the experimental pictures according to the number of Rydberg excitations they display allowed the researchers to reveal geometric patterns in the arrangement of the Rydberg excitations. (a) Individual snapshots. (b) Spatial distribution averaged over several hundreds of snapshots.” Credit: MPI of Quantum Optics
Normally, only those rubidium atoms that lie in the same dimple of the egg carton interact with one another. However, it would be important for quantum information processing that interactions be possible over longer distances within the atomic ensemble so that – analogous to a transistor – the state of one atom can switch that of another Atoms in a Rydberg state do provide such interactions, as the researchers based in Garching have now illustrated by observing the spatial arrangement of the Rydberg excitations. “Individual atoms in the gas, however, were not excited to Rydberg atoms in the experiment; each Rydberg excitation was distributed over several atoms in accordance with the laws of quantum mechanics. This collective excitation behaves like a well-defined particle encompassing many atoms, like a ‘super atom’ in a way.
Several Rydberg excitations interact with one another at a distance
Peter Schauß and his colleagues had to create several Rydberg excitations in the atomic ensemble in order to observe a long-range interaction. They succeeded by prolonging the pulse duration of the laser excitation. They finally determined the number and position of the ‘super atoms’ using a measurement technique developed in the group. Roughly speaking, they caused the excitations to glow by exciting them with a laser beam of suitable frequency and then determined the location of this luminescence by simply taking a picture of it with a highly sensitive digital camera.
The researchers thereby observed that the super atoms were behaving as though they were avoiding one another: When they created two or more Rydberg excitations in the atomic ensemble, these always appeared with a minimum separation between them. The reason for this mutual blockade is what is known as van der Waals forces. These forces arise between two atoms or molecules through fluctuations of electrical charges in their electron shells, during which electrical plus and minus poles temporarily arise. The van der Waals forces are mostly attractive, but can also be repulsive. In the Garching experiment, the latter was the case. A kind of blockade radius resulted from this, which made the ‘super atoms’ behave to a certain extent like hard spheres that do not permeate one another.” It was really exciting when three or more of these ‘super atoms’ arose in the atomic ensemble,” says Peter Schauß. Three Rydberg excitations ordered themselves into equilateral triangles, four into squares, and five into regular pentagons. How these geometric shapes were oriented in the plane of the optical lattice varied, however. “In each picture, the polygon was oriented in a random way.”
Only a quantum mechanical many-body system is useful for quantum computation.
“The ‘super atoms’ behaved according to the fundamental tendency of nature to form ordered and compact structures,” explained Peter Schauß. This principle leads, for instance, to the atoms or molecules coming together in crystals with regular structure. As a consequence, the researchers in Garching observed something close to Rydberg crystals. “That is an exotic phase of matter which has never been directly observed before,” says physicist Marc Cheneau, who participated in the experiment.
Following this discovery, the scientists investigated whether the geometric patterns of Rydberg excitations that they observed could be described by classical mechanics or only by quantum mechanics. In a classical sense, three Rydberg excitations would arrange into a triangle with a well-defined orientation, but this orientation would vary each time we reproduce the experiment. In the quantum sense, the triangle would be simultaneously in all possible orientations until we make the Rydberg atoms glow. “This question is important to resolve, since quantum computers will have to utilize the full power of quantum mechanics. A system containing Rydberg excitations would thus only be useful if the latter form a quantum mechanical many-body state. “The better we physicists can control such quantum mechanical systems, the sooner we can develop a high-performance quantum computer,” says Marc Cheneau.
The objective is more control over the Rydberg excitations
Should the researchers in Garching actually have created a quantum mechanical many-body system, the state of the system arises from a superposition of the geometric patterns observed with the various numbers of Rydberg excitations and/or various orientations in the plane
Peter Schauß and his colleagues discovered a clear indication that the Rydberg excitations formed a quantum mechanical many-body state. They measured how the average number of Rydberg excitations in the gas of rubidium atoms depended on the duration of the laser pulse excitation. The dynamics appeared to be 10 times faster than expected for a classical state, but in good agreement with a model assuming a quantum state. This is encouraging, but not yet a definite proof for the pure quantum mechanical nature of the geometric patterns. As a next step, the researchers in Garching intend to create a real Rydberg crystal with a well-defined number of Rydberg excitations. Peter Schauß thinks that may be a real challenge. “The objective is to attain complete control over the quantum mechanical many-body system,” says the physicist. To carry out complex operations with a quantum computer, it may be necessary to control as many Rydberg excitations as possible. In the long term, mastering Rydberg gases could facilitate the construction of a scalable system for quantum information processing, i.e. a system that can be expanded without large additional effort and cost.
Reference: “Observation of spatially ordered structures in a two-dimensional Rydberg gas” by Peter Schauß, Marc Cheneau, Manuel Endres, Takeshi Fukuhara, Sebastian Hild, Ahmed Omran, Thomas Pohl, Christian Gross, Stefan Kuhr and Immanuel Bloch, 31 October 2012, Nature.
DOI: 10.1038/nature11596
I impressed by the quality of information on this website. I am very enjoyed for this blog. Its an informative topic. Very useful info. I am sure I will visit this place again soon. Hope to see more posts soon!