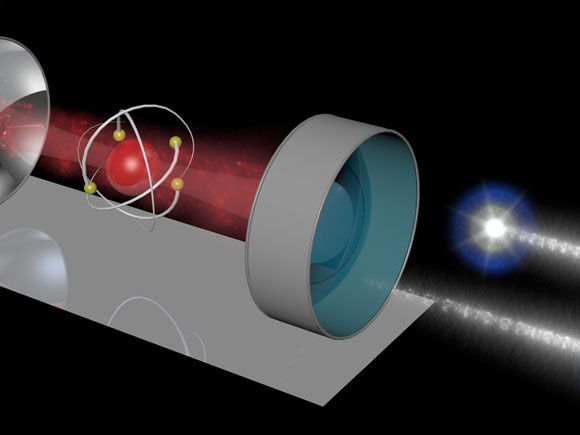
A universal quantum gate: Max Planck physicists make two photons (right) interact with each other by using an atom in a resonator as a mediator. The resonator consists of two mirrors with a laser trapping the atom between them. Credit: Stephan Welte/MPI of Quantum Optics
Scientists have now developed a universal quantum gate, which could become the key component in a quantum computer.
Light particles completely ignore each other. In order that these particles can nevertheless switch each other when processing quantum information, researchers at the Max Planck Institute of Quantum Optics in Garching have now developed a universal quantum gate. Quantum gates are essential elements of a quantum computer. Switching them with photons, i.e. light particles, would have practical advantages over operating them with other carriers of quantum information.
The light-saber fights of the Jedi and Sith in the Star Wars saga may well suggest something different, but light beams do not notice each other. No matter how high their intensity, they cut through each other without hindrance. When individual light particles meet, as is necessary for some applications of quantum information technology, nothing at all happens. Photons can therefore not switch each other just like that, as would have to be the case if one wanted to use them to operate a quantum gate, the elementary computing unit of a quantum computer.
A quantum computer can master some tasks, such as searching through databases, much faster than conventional computers. Physicists have already developed quantum gates for the super-computers of the future, for example by using nitrogen atoms contained in diamonds as impurities as the smallest computing unit. But “to have a quantum computer compute with photons would have practical advantages,” says Stephan Ritter, who leads a Research Group in Gerhard Rempe’s Division at the Max Planck Institute of Quantum Optics. “This is because quantum information has to be in the form of photons in order to be transmitted over large distances. If we can use photons to process it as well, we do not have to transfer it to other carriers, such as atoms, in order to compute with it.”
An atom in a resonator mediates between light particles
In order for photons to sense each other’s presence in the first place, let alone switch each other, they need mediators. In the experiments being conducted by Stephan Ritter’s team of physicists, this mediating role is taken on by a single atom in a resonator. The resonator consists of two mirrors 0.5 mm apart. The Garching-based researchers use a laser beam to trap the atom in the resonator.
For their experiments, the scientists now need two photons each carrying one qubit. A qubit is the quantum mechanical equivalent of the bit of a conventional computer. It can, however, not only encode the zero and the one, but assume all possible states in between as well. The researchers write the states of the two qubits into the polarization of the two light particles, i.e. into the direction of oscillation of the electromagnetic waves.
The Max Planck physicists send the two photons, one shortly after the other, onto the system of atoms and resonator. The first photon thereby transfers information to the atom by changing its state – but only if the photon has the right polarization. This change then has an effect on the polarization of the second photon when it impinges onto the system of atom and resonator a short time later.
The quantum gate operates in a deterministic way
“Our system only becomes a universal quantum gate because the second photon can also transfer information onto the first photon, however,” says Bastian Hacker, who conducted the experiments as part of his doctoral thesis. To this end, the scientists initially store the two photons in an optical fiber more than one kilometer in length after the light particles have been reflected at the resonator. At the same time, they conduct a measurement on the atom, which can also affect the polarization state of the two photons due to the surprising properties of quantum mechanics. As is the case with a conventional bit, there are only two possible measurement results. They provide the researchers with reliable information about which rotation of the polarization of the first photon they can use to complete the gate operation.
“Our quantum gate operates in a deterministic way,” says Stephan Ritter. This means that the scientists can reliably predict which changes the light particles should experience in the quantum gate depending on the original polarization of the photons fed in. In addition, the gate carries out these operations on all photons which impinge on the resonator with the trapped atom – at least in principle. In reality, unavoidable technical shortcomings decrease the efficiency of the quantum gate as well as the precision of its operations. However, the researchers already have some ideas about how they can improve the two characteristics of the quantum gate: by using mirrors with lower losses, for example, or a storage device for the photons which is more efficient than an optical fiber. In other implementations of quantum gates between photons with which physicists have already experimented, the errors are inherent, however, because chance always plays a role here.
Two experiments demonstrate how reliable the quantum gate is
The Garching-based researchers have conducted two experiments to demonstrate how reliably their quantum gate already operates. Which operations the quantum gate executes here depends only on how the two input photons are polarized.
In one experiment, the researchers circularly polarize the first photon so that its direction of oscillation rotates either clockwise or counter-clockwise. The second photon is linearly polarized, i.e. so that it oscillates in a horizontal or vertical plane. On a photon pair with these input states, the quantum gate acts like a CNOT operation, where the first qubit controls the second one. This is because, depending on the direction in which the first photon rotates, the quantum gate flips the polarization of the second photon – from the vertical to the horizontal plane, for example – or not. CNOT gates are essential for a quantum computer, because they can be used to execute all logic operations.
For the second experiment, the researchers in Garching polarize both photons linearly. Fed with such input states, the quantum gate entangles the two photons. Entangled photons can no longer be described independently of each other, but only with a common state – no matter how great the distance between the two light particles. As much as entanglement puts our imagination to the test, for the quantum computer it is an indispensable ingredient like the CNOT gate. “Only the entanglement of qubits allows the strength of the quantum computer to be unfolded,” says Stephan Welte, who also contributed crucial work to the experiments as part of his doctoral thesis.
The atom in the resonator as the key element of a quantum computer
“With the quantum gate, we now have a key element for an optical quantum computer,” says Gerhard Rempe, Director at the Max Planck Institute in Garching. It will be a while before such a quantum computer completes some computing tasks at a speed which will outclass any conventional computer, however; not least because this requires the quantum gate to compute more reliably. Nevertheless, Gerhard Rempe already has definite ideas about how such a super-computer could be operated with an atom in the resonator. This would not require many of these systems, each of which can quite easily fill a laboratory. “The logic operations can be carried out one after the other with a single atom in a resonator,” says Gerhard Rempe.
The European Commission obviously also believes that these quantum technology concepts have a future. It plans to invest one billion euros into its development over a period of approx. ten years. This funding could also speed up the process of realizing the superfast quantum computer – which is also what Stephan Ritter and his colleagues in Garching are hoping for.
Reference: “A photon-photon quantum gate based on a single atom in an optical resonator” by Bastian Hacker, Stephan Welte, Gerhard Rempe and Stephan Ritter, 6 July 2016, Nature.
DOI: 10.1038/nature18592
arXiv:1605.05261
How can anything be completely ignored and yet have interaction(?).
“It can, however, not only encode the zero and the one, but assume all possible states in between as well.” What is between 1 and zero? Or what states is it referring?
It can be in “superposition ” both on and off… state 1, 0 and both. Just as particles are only in a given position when we observe them.
With only 0 or 1, we have a base-2 digital system, the binary code. For example, 2^10 = 1024, which is the digital Kilo. With 0, 1, or any fraction in between, we have a base-infinity digital system. Of course, in real life, we can only apply a finite base digital system, that would be done by picking whatever desired fractions in between 0 and 1 along with 0 and 1.
One should always remember the observer is a wave function with quantum interaction. The observer interacts at quantum level with the observed.
Well said Primordial.. well said… This is nuts… its amazing… i cant wait to see the power of a 64 qbit system when completed… GAME CHANGER
The CNOT Quantum Gate would be the counterpart of the NAND Gate (Inverter) in conventional/classical computers.
Hydrogen. Water or crystal sound vibration lubricated
It will be a while before such a quantum computer completes some computing tasks at a speed which will outclass any conventional computer. ……How much can be here « a while « ?….in realistic macroscopic time , or not quantic terms ?