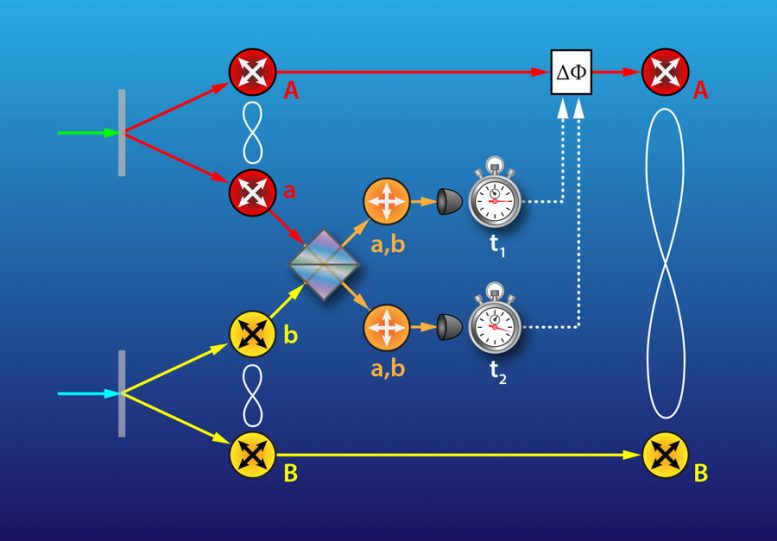
Figure 1. To entangle independent photons of different colors, researchers use down-conversion to generate two pairs of polarization-entangled photons, labeled (A, a) and (B, b). The frequencies of each pair differ by either 40 or 80 MHz. Photons a and b enter a beam splitter, resulting in a “hypoentangled” state of polarization and frequency. Two detectors record the arrival times, t1 and t2, of the two photons, and this information is fed forward to a device that applies a time-dependent phase shift to photon A. In the end, the entanglement of a and bis swapped to A and B. Credit: APS/Alan Stonebraker
Using time-resolved measurement and active feed forward, researchers from the University of Science and Technology of China have entangled two independent photons of different colors for the first time.
Entanglement has been capturing the imagination of scientists as well as the public ever since it first emerged from the newly minted quantum mechanics formalism eight decades ago. Two particles are considered entangled when their states cannot be described separately, thus allowing counterintuitive action-at-a-distance whenever one particle is measured. Entanglement powers certain quantum information technologies that ultimately promise to outperform classical devices. The prerequisite to engineering quantum processors, simulators, and sensors is our ability to generate entanglement between large numbers of quantum systems such as photons. Typically, this entanglement-generation requires that the photons be indistinguishable. However, a new experiment described in Physical Review Letters manages, for the first time, to entangle independently created photons that have different frequencies, or colors [1]. The results could be useful for entangling photons from arrays of nonidentical quantum dots, which often emit photons with slightly different frequencies.
Photons are often entangled at their creation. For example, pairs of identical photons can be generated by “down-converting” single pump photons in a nonlinear crystal. Unfortunately, this process is inefficient because the down-conversion has a low probability. One alternative is to entangle photons coming from different sources. This can be done by sending two photons into a beam splitter and taking advantage of the quantum phenomenon of two-photon interference: If an observer behind the beam splitter cannot tell which path each individual photon took, their probabilistic outcomes can add up or cancel out, leaving those photons that exit the beam splitter through different ports in an entangled state. This effect has been the workhorse of the quantum photonics community for decades [2].
To achieve perfect interference, the photons involved must be indistinguishable when they are eventually measured. However, contrary to common intuition, they don’t necessarily have to be indistinguishable when they hit the beam splitter; they may well have very different properties—different color, polarization, spatial mode, etc.,—and yet we can still recover interference if our detectors are insensitive to those properties, or if the respective information is “erased” before detection. Tiang-Ming Zhao and colleagues from the University of Science and Technology of China in Hefei have developed a clever method to erase frequency information, so that photons from distinct sources and of different color can become entangled through their interference [1].
The heart of their idea works as follows: two polarization-encoded photons with slightly different frequencies are sent into a polarizing beam splitter. If the photons were indistinguishable, the output would be entangled (assuming the photons leave from different ports). But the photons in Zhao et al.’s experiment can of course be distinguished by their color. In consequence, the beam splitter will not output the photons in a clean polarization-entangled state but rather in what has sometimes been called a “hypoentangled” state [3], in which polarization and color are intrinsically linked to each other. If one tried to measure either the color or the polarization independently, the remaining state would end up being mixed, i.e., not entangled. One can however try to carefully tease out 1 degree of freedom selectively and keep the entanglement alive in the other degree.
This technique has been applied before in a very similar scenario involving color and polarization by my colleagues and me [3]. In this previous case, we subjected our hypoentangled state to a joint polarization projection, and the output was a discretely color-entangled two-photon state. Here, in contrast, Zhao et al. erase the color information, leaving the eventual state entangled in polarization. They do this by making high-resolution time measurements at the outputs of their beam splitter. Time is related to frequency, and the frequency information in the incoming state can just as well be interpreted as timing information. If we simply averaged over the arrival times of the two photons behind the beam splitter indiscriminately, the remaining state would be mixed. However, the authors show that picking a certain timing combination allows the remaining state to stay intact and indeed be entangled in polarization. The researchers’ timing protocol effectively erases the frequency-distinguishing information about the photons and allows them to create a final state that is entangled up to a time-dependent phase factor that can be corrected for.
One problem is that the frequency eraser measures the photons, so they are no longer available for evaluation of their polarization entanglement. To get around this, the researchers included an entanglement-swapping protocol [4] that transfers the entanglement to two other photons, which can be subsequently evaluated. Specifically, their experiment starts with the down-conversion of two pump beams that each produce a pair of polarization-entangled photons with wavelengths around 795 nanometers (see Fig. 1). The first pair, labeled A and a, differs from the second pair, labeled B and b, by a frequency of either 40 or 80 megahertz (MHz), which is 8 to 16 times the linewidth of the photons. The team sends photons a and b into a beam splitter to produce a hypoentangled state of polarization and frequency. The frequency-distinguishing information is erased by two photon detectors on the far side of the beam splitter. The photon arrival times, t1 and t2, are forwarded to an optical component that adds a time-dependent phase shift to photon A. As a result, the polarization entanglement between a and b is transferred with high fidelity to A and B, as verified by the authors with polarization measurements of A and B.
The results could be of interest in efforts to build scalable devices from sophisticated photon sources such as quantum dots. Quantum dots can generate photons virtually deterministically, but they do suffer from the drawback that dots cannot be created entirely identical and hence create photons with slightly different spectral characteristics. It would be very exciting indeed if temporal quantum erasure could be used to create large-scale entangled clusters of photons emitted from dissimilar quantum dots.
It is still a long way to go from here, though, as the current scheme will face many challenges before it becomes practicable. The main concern is whether it will be able to entangle photons with an efficiency of at least 1/2—the rule-of-thumb threshold required for scalability of photonic architectures. Another issue is that the finite temporal resolution of the photon detectors constrains the maximal spectral separation that can be erased in the time-resolved two-photon interference measurement. For the best available conventional photon detectors, the allowed spectral separation is on the order of MHz. Therefore, one would need quantum dots that emit photons within a few MHz of each other. Current dot fabrication is starting to reach that level of precision, but engineers may well remove the remaining indistinguishability between sources by external tuning, via temperature or electric field controls [5]. What is perhaps a more interesting venue for the research presented here is its fundamental angle, and in particular the possibility of applying more sophisticated temporal filters in the detection, which might be useful for quantum state engineering.
References:
- “Entangling Different-Color Photons via Time-Resolved Measurement and Active Feed Forward” by Tian-Ming Zhao, Han Zhang, Jian Yang, Zi-Ru Sang, Xiao Jiang, Xiao-Hui Bao and Jian-Wei Pan, 10 March 2014, Physical Review Letters.
DOI: 10.1103/PhysRevLett.112.103602 - “Measurement of subpicosecond time intervals between two photons by interference” by C. K. Hong, Z. Y. Ou and L. Mandel, 2 November 1987, Physical Review Letters.
DOI: 10.1103/PhysRevLett.59.2044 - “Discrete Tunable Color Entanglement” by S. Ramelow, L. Ratschbacher, A. Fedrizzi, N. K. Langford and A. Zeilinger, 16 December 2009, Physical Review Letters.
DOI: 10.1103/PhysRevLett.103.253601 - “Teleporting an unknown quantum state via dual classical and Einstein-Podolsky-Rosen channels” by Charles H. Bennett, Gilles Brassard, Claude Crépeau, Richard Jozsa, Asher Peres and William K. Wootters, 29 March 1993, Physical Review Letters.
DOI: 10.1103/PhysRevLett.70.1895 - “Two-photon interference of the emission from electrically tunable remote quantum dots” by Raj B. Patel, Anthony J. Bennett, Ian Farrer, Christine A. Nicoll, David A. Ritchie and Andrew J. Shields, 11 July 2010, Nature Photonics.
DOI: 10.1038/nphoton.2010.161
What kind of protection can I get to block microwave weapons high power ionizing radiation from cyberspace.strangulation and .