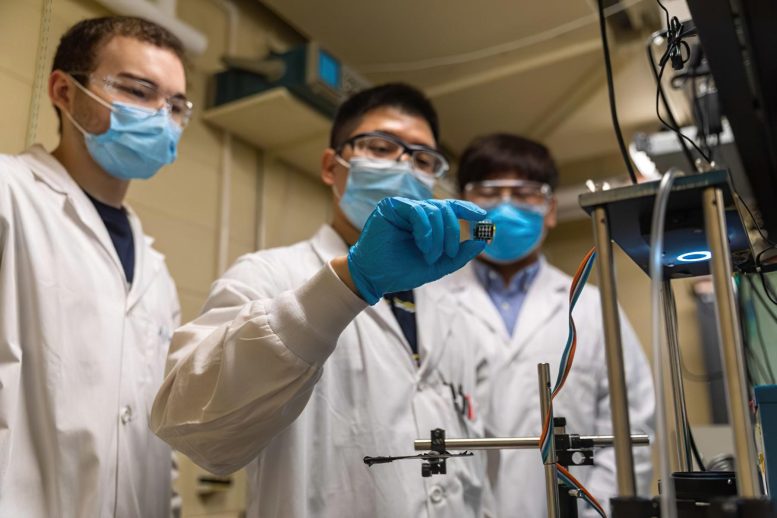
Northwestern University’s latest research in perovskite solar cells has set a new efficiency record of 25.1%, using a novel dual-molecule approach to reduce electron recombination. This development marks a significant step towards making perovskite solar cells a more efficient and stable alternative to conventional silicon-based cells. Credit: Sargent Lab/Northwestern University
Researchers have enhanced cell efficiency by utilizing a combination of molecules to tackle various issues.
Researchers at Northwestern University have once again elevated the standards for perovskite solar cells with a new development that helped the emerging technology hit new records for efficiency.
The findings, recently published in the journal Science, describe a dual-molecule solution to overcoming losses in efficiency as sunlight is converted to energy. By incorporating first, a molecule to address something called surface recombination, in which electrons are lost when they are trapped by defects — missing atoms on the surface, and a second molecule to disrupt recombination at the interface between layers, the team achieved a National Renewable Energy Lab (NREL) certified efficiency of 25.1% where earlier approaches reached efficiencies of just 24.09%.
Focusing on Interfacial Recombination
“Perovskite solar technology is moving fast, and the emphasis of research and development is shifting from the bulk absorber to the interfaces,” said Northwestern professor Ted Sargent. “This is the critical point to further improve efficiency and stability and bring us closer to this promising route to ever-more-efficient solar harvesting.”
Sargent is the co-executive director of the Paula M. Trienens Institute for Sustainability and Energy (formerly ISEN) and a multidisciplinary researcher in materials chemistry and energy systems, with appointments in the department of chemistry in the Weinberg College of Arts and Sciences and the department of electrical and computer engineering in the McCormick School of Engineering.
Conventional solar cells are made of high-purity silicon wafers that are energy-intensive to produce and can only absorb a fixed range of the solar spectrum.
Perovskite materials whose size and composition can be adjusted to “tune” the wavelengths of light they absorb, making them a favorable and potentially lower-cost, high-efficiency emerging tandem technology.
Historically perovskite solar cells have been plagued by challenges to improve efficiency because of their relative instability. Over the past few years, advances from Sargent’s lab and others have brought the efficiency of perovskite solar cells to within the same range as what is achievable with silicon.
Advancements in Electron Retention
In the present research, rather than trying to help the cell absorb more sunlight, the team focused on the issue of maintaining and retaining generated electrons to increase efficiency. When the perovskite layer contacts the electron transport layer of the cell, electrons move from one to the other. But the electron can move back outward and fill, or “recombine” with holes that exist on the perovskite layer.
“Recombination at the interface is complex,” said first author Cheng Liu, a postdoctoral student in the Sargent lab, which is co-supervised by the Charles E. and Emma H. Morrison Professor of Chemistry Mercouri Kanatzidis. “It’s very difficult to use one type of molecule to address complex recombination and retain electrons, so we considered what combination of molecules we could use to more comprehensively solve the problem.”
Past research from Sargent’s team has found evidence that one molecule, PDAI2, does a good job at solving interface recombination. Next, they needed to find a molecule that would work to repair surface defects and prevent electrons from recombining with them.
Dual-Molecule Approach and Future Work
By finding the mechanism that would allow PDAI2 to work with a secondary molecule, the team narrowed in on sulfur, which could replace carbon groups — typically poor at preventing electrons from moving — to cover missing atoms and suppress recombination.
A recent paper by the same group published in Nature developed a coating for the substrate beneath the perovskite layer to help the cell work at a higher temperature for a longer period. This solution, according to Liu, can work in tandem with the findings within the Science paper.
While the team hopes their findings will encourage the larger scientific community to continue moving the work forward, they too will be working on follow-ups.
“We have to use a more flexible strategy to solve the complex interface problem,” Cheng said. “We can’t only use one kind of molecule, as people previously did. We use two molecules to solve two kinds of recombination, but we are sure there’s more kinds of defect-related recombination at the interface. We need to try to use more molecules to come together and make sure all molecules work together without destroying each other’s functions.”
Reference: “Bimolecularly passivated interface enables efficient and stable inverted perovskite solar cells” by Cheng Liu, Yi Yang, Hao Chen, Jian Xu, Ao Liu, Abdulaziz S. R. Bati, Huihui Zhu, Luke Grater, Shreyash Sudhakar Hadke, Chuying Huang, Vinod K. Sangwan, Tong Cai, Donghoon Shin, Lin X. Chen, Mark C. Hersam, Chad A. Mirkin, Bin Chen, Mercouri G. Kanatzidis and Edward H. Sargent, 16 November 2023, Science.
DOI: 10.1126/science.adk1633
The paper was supported under award number 70NANB19H005 from the US Department of Commerce, National Institute of Standards and Technology, as part of the Center for Hierarchical Materials Design (CHiMaD), and partially by OSR-CRG2020-4350.2, in addition to receiving support from the Office of Naval Research (N00014-20-1-2572, N00014-20-1-2725), the Army Research Office (W911NF-23-1-0141, W911NF-23-1-0285, and by the Sherman Fairchild Foundation, Inc.). The work made use of the SPID, EPIC, and Keck-II facilities of Northwestern University’s NUANCE Center, which has received support from the SHyNE Resource (NSF ECCS-2025633), The International Institute of Nanotechnology, Northwestern University and Northwestern’s 5 MRSEC program (NSF DMR-1720139). Charge transport characterization was supported by the National Science Foundation (NSF) Materials Research Science and Engineering Center at Northwestern University (DMR-1720319.)
“Perovskite” is an internationally approved name for a mineral with a defined chemical composition and structure. What is being described in this article is something with a perovskite-like structure, but a very different composition. It is an important distinction! Researchers should not be so careless with their nomenclature because it can lead to confusion.
https://www.mindat.org/min-3166.html
Might I suggest that they be referred to as PLS (Perovskite-Like Structure) solar cells because their principle similarity is the crystal lattice.
Well…took me all of 1 google search to learn that “perovskite” is indeed the name of not just a specific mineral, but also any compound with that crystal structure.
So turns out he was not being careless. He was using completely correct accepted terminology.
Just because some materials scientists use a term casually or carelessly doesn’t mean it is correct or a good idea. It just shows that they are not specialists in mineralogy. They don’t know what they don’t know.
It appears that you didn’t read the link I provided. What is your source that you are using to support the idea that any compound that is isostructural with perovskite is correctly called the same as the recognized mineral?
The International Mineralogical Association (IMA) has the accepted authority to name new minerals, and to revoke official usage that is either a different mineral or misidentified. Consider the potential confusion if one applied the results of convergent evolution that have produced dolphin-like reptiles and simply called them “dolphins” because they looked similar to modern mammals.
It also appears that you missed a subtlety. The IMA recognizes perovskite subgroups and supergroups, including synthetic compounds. However, if the synthetic material is not found in nature (non-synthetic) then it doesn’t meet the definition of being a mineral. So, you are advocating that a true mineral with a specific approved name be used interchangeably with non-minerals. Furthermore, you are advocating that the long-held practice of compounds that are isostructural, but chemically quite dissimilar, be given different names to distinguish them from each other, or at least placed in a ‘group’ that is isostructural.
I maintain that using the accepted name of a mineral to identify a non-mineral whose principle commonality is a similar structure leads to the potential of confusion, and to prevent that confusion, more words are then required to note the important differences. The reason that English has so many words is precisely to provide a succinct and non-ambiguous identification of things. That is especially true in science, where precision is valued more highly than in common speech.
It takes more than a quick search on the internet, and finding one link written by some unknown person that ‘thinks’ as you do, to be an expert.