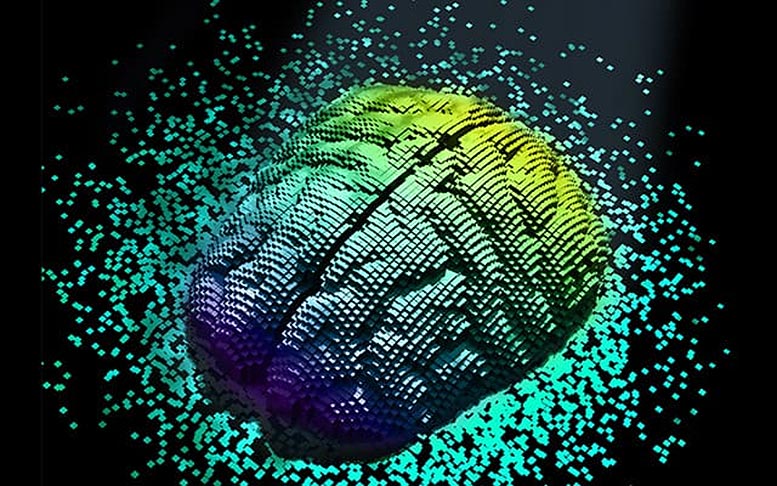
The development of the fetal brain involves the creation and migration of billions of neurons during the course of pregnancy. Credit: Veronika Mertens
Researchers track the cellular migration of developing fetal brains for the first time by backtracking genetic mutations documented in deceased adult brains.
The development of a human brain remains a mostly mysterious process that races from an embryonic neural tube and ends with more than 100 billion interconnected neurons in the brain of a newborn. To achieve this marvel of biological engineering, the developing fetal brain must grow, on average, at a rate of approximately 250,000 nerve cells per minute throughout the course of a pregnancy.
These nerve cells are frequently created far from where they will eventually reside and function in the new brain, a migration that has been extensively researched in animal models using chemical or biological tracers but has never been directly studied in humans. That is, until now.
In a new paper, published online on April 20, 2022, in the journal Nature, scientists at University of California San Diego (UCSD) School of Medicine and Rady Children’s Institute of Genomic Medicine describe novel methods for inferring the movement of human brain cells during fetal development by studying healthy adult individuals who have recently passed away from natural causes.
“Every time a cell divides into two daughter cells, by chance, there arise one or more new mutations, which leave a trail of breadcrumbs that can be read out by modern DNA sequencers,” said senior author Joseph Gleeson, MD, Rady Professor of Neuroscience at UC San Diego School of Medicine and director of neuroscience research at the Rady Children’s Institute for Genomic Medicine.
“By developing methods to read these mutations across the brain, we are able to reveal key insights into how the human brain forms, in comparison with other species.”
The structure of the human neocortex underlies species-specific traits and reflects intricate developmental programs. Here we sought to reconstruct processes that occur during early development by sampling adult human tissues. We analyzed neocortical clones in a post-mortem human brain through a comprehensive assessment of brain somatic mosaicism, acting as neutral lineage recorders. We combined the sampling of 25 distinct anatomic locations with deep whole-genome sequencing in a neurotypical deceased individual and confirmed results with five samples collected from each of three additional donors. We identified 259 bona fide mosaic variants from the index case, then deconvolved distinct geographical, cell-type and clade organizations across the brain and other organs. We found that clones derived after the accumulation of 90–200 progenitors in the cerebral cortex tended to respect the midline axis, well before the anterior–posterior or entral–dorsal axes, representing a secondary hierarchy following the overall patterning of forebrain and hindbrain domains. Clones across neocortically derived cells were consistent with a dual origin from both dorsal and ventral cellular populations, similar to rodents, whereas the microglia lineage appeared distinct from other resident brain cells. Our data provide a comprehensive analysis of brain somatic mosaicism across the neocortex and demonstrate cellular origins and progenitor distribution patterns within the human brain.
Although there are 3 billion DNA bases — and more than 30 trillion cells in the human body — Gleeson and colleagues focused their efforts on just a few hundred DNA mutations that likely arose during the first few cell divisions after fertilization of the embryo or during early development of the brain. By tracking these mutations throughout the brain in deceased individuals, they were able to reconstruct development of the human brain for the first time.
To understand the type of cells displaying these breadcrumb mutations, they developed methods to isolate each of the major cell types in the brain. For instance, by profiling the mutations in excitatory neurons compared with inhibitory neurons, they confirmed the long-held suspicion that these two cell types are generated in different germinal zones of the brain, and then later mix together in the cerebral cortex, the outermost layer of the organ.
However, they also discovered that the mutations found in the left and right sides of the brain were different from one another, suggesting that — at least in humans — the two cerebral hemispheres separate during development much earlier than previously suspected.
The results have implications for certain human diseases, like intractable epilepsies, where patients show spontaneous convulsive seizures and require surgery to remove an epileptic brain focus, said Martin W. Breuss, PhD, former project scientist at UC San Diego and now an assistant professor at the University of Colorado School of Medicine.
Breuss is co-first author with Xiaoxu Yang, PhD, postdoctoral scholar, and Johannes C. M. Schlachetzki, MD, project scientist, both at UC San Diego; and Danny Antaki, PhD, a former postdoctoral scholar at UC San Diego, now at Twist Biosciences.
“This study,” the authors said, “solves the mystery as to why these foci are almost always restricted to one hemisphere of the brain. Applying these results to other neurological conditions could help scientists understand more mysteries of the brain.”
Reference: “Somatic mosaicism reveals clonal distributions of neocortical development” by Martin W. Breuss, Xiaoxu Yang, Johannes C. M. Schlachetzki, Danny Antaki, Addison J. Lana, Xin Xu, Changuk Chung, Guoliang Chai, Valentina Stanley, Qiong Song, Traci F. Newmeyer, An Nguyen, Sydney O’Brien, Marten A. Hoeksema, Beibei Cao, Alexi Nott, Jennifer McEvoy-Venneri, Martina P. Pasillas, Scott T. Barton, Brett R. Copeland, Shareef Nahas, Lucitia Van Der Kraan, Yan Ding, NIMH Brain Somatic Mosaicism Network, Christopher K. Glass and Joseph G. Gleeson, 20 April 2022, Nature.
DOI: 10.1038/s41586-022-04602-7
Co-authors include: Xin Xu, Changuk Chung, Guoliang Chai, Valentina Stanley, Qiong Song, Traci F. Newmeyer, An Nguyen, Beibei Cao, Jennifer McEvoy-Venneri and Brett R. Copeland, all at UC San Diego and Rady Children’s Institute for Genomic Medicine; Addison J. Lana, Sydney O’Brien, Marten A. Hoeksema, Alexi Nott, Martina P. Pasilla, Scott T. Barton, and Christopher K. Glass, all at UC San Diego; Shareef Nahas, Lucitia Van Der Kraan and Yan Ding, Rady Children’s Institute for Genomic Medicine and the NIMH Brain Somatic Mosaicism Network.
Funding for this research came, in part, from the Howard Hughes Medical Institute, the National Institute of Mental Health (grants MH108898, RO1 MH124890, R21 AG070462), the National Institute on Aging (grants RF1 AGO6106-02, R01 AGO56511-02, R01 NS096170-04) and the UC San Diego IGM Genomics Center (S10 OD026929).
Be the first to comment on "Where Neurons Begin in the Brain Isn’t Necessarily Where They End"