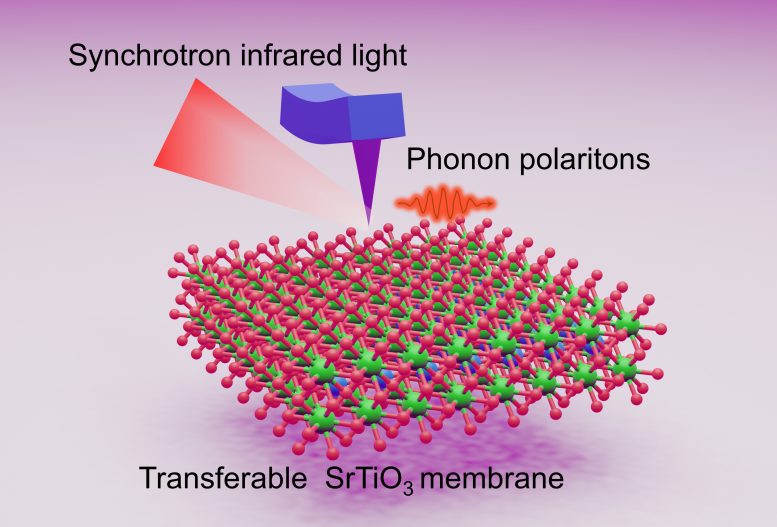
Researchers have demonstrated that a specific class of oxide membranes can confine infrared light much more effectively than bulk crystals, which has promising implications for next-generation infrared imaging technologies. These thin-film membranes maintain the desired infrared frequency while compressing wavelengths, allowing for greater image resolution. Using transition metal perovskite materials and advanced synchrotron near-field spectroscopy, the researchers showed that phonon polaritons in these membranes can confine infrared light to just 10% of its wavelength. This breakthrough could lead to new applications in photonics, sensors, and thermal management, with potential ease of integration into various devices. Credit: Yin Liu, NC State University
A new study reveals that oxide membranes can confine infrared light to a greater extent than traditional methods, promising advancements in imaging resolution and applications in photonics and thermal management.
Researchers have successfully shown that a particular type of oxide membranes can effectively confine, or “squeeze,” infrared light. This breakthrough could enhance future infrared imaging technologies. These thin-film membranes outperform traditional bulk crystals in confining infrared light.
“The thin-film membranes maintain the desired infrared frequency, but compress the wavelengths, allowing imaging devices to capture images with greater resolution,” says Yin Liu, co-corresponding author of a paper on the work and an assistant professor of materials science and engineering at North Carolina State University.
“We’ve demonstrated that we can confine infrared light to 10% of its wavelength while maintaining its frequency – meaning that the amount of time that it takes for a wavelength to cycle is the same, but the distance between the peaks of the wave is much closer together. Bulk crystal techniques confine infrared light to around 97% of its wavelength.”
Experimental Breakthroughs in Thin-Film Technology
“This behavior was previously only theorized, but we were able to demonstrate it experimentally for the first time through both the way we prepared the thin-film membranes and our novel use of synchrotron near-field spectroscopy,” says Ruijuan Xu, co-lead author of the paper and an assistant professor of materials science and engineering at NC State.
For this work, the researchers worked with transition metal perovskite materials. Specifically, the researchers used pulsed laser deposition to grow a 100-nanometer-thick crystalline membrane of strontium titanate (SrTiO3) in a vacuum chamber. The crystalline structure of this thin film is high quality, meaning that it has very few defects. These thin films were then removed from the substrate they were grown on and placed on the silicon oxide surface of a silicon substrate.
The researchers then made use of the technology at the Advanced Light Source of the Lawrence Berkeley National Laboratory to perform synchrotron near-field spectroscopy on the strontium titanate thin film as it was exposed to infrared light. This enabled the researchers to capture the interaction of the material with infrared light at the nanoscale.
Understanding Energy Transfer: Phonons, Photons, and Polaritons
To understand what the researchers learned, we need to talk about phonons, photons, and polaritons. Phonons and photons are both ways that energy travels through and between materials. Phonons are essentially the waves of energy caused by how atoms vibrate. Photons are essentially the waves of electromagnetic energy. You can think of phonons as units of sound energy, whereas photons are units of light energy. Phonon polaritons are quasi-particles that occur when an infrared photon is coupled with an “optical” phonon – meaning a phonon that can emit or absorb light.
“Theoretical papers proposed the idea that transition metal perovskite oxide membranes would allow phonon polaritons to confine infrared light,” Liu says. “And our work now demonstrates that the phonon polaritons do confine the photons, and also keep the photons from extending beyond the surface of the material.
“This work establishes a new class of optical materials for controlling light in infrared wavelengths, which has potential applications in photonics, sensors, and thermal management,” Liu says. “Imagine being able to design computer chips that could use these materials to shed heat by converting it into infrared light.”
“The work is also exciting because the technique we’ve demonstrated for creating these materials means that the thin films can be easily integrated with a wide variety of substrates,” Xu says. “That should make it easy to incorporate the materials into many different types of devices.”
Reference: “Highly confined epsilon-near-zero and surface phonon polaritons in SrTiO3 membranes” by Ruijuan Xu, Iris Crassee, Hans A. Bechtel, Yixi Zhou, Adrien Bercher, Lukas Korosec, Carl Willem Rischau, Jérémie Teyssier, Kevin J. Crust, Yonghun Lee, Stephanie N. Gilbert Corder, Jiarui Li, Jennifer A. Dionne, Harold Y. Hwang, Alexey B. Kuzmenko and Yin Liu, 4 June 2024, Nature Communications.
DOI: 10.1038/s41467-024-47917-x
The research was done with support from the U.S. Department of Energy, Office of Basic Energy Sciences, Division of Materials Sciences and Engineering, under contract no. DE-AC02-76SF00515; and by the National Science Foundation under grant number 2340751.
Be the first to comment on "“Previously Only Theorized” – Researchers Demonstrate New Way To “Squeeze” Infrared Light"