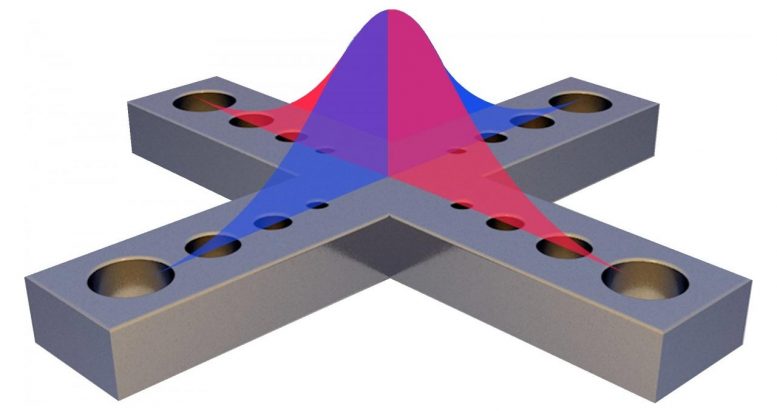
A design for the photonic structure that traps two photons. Photons travel in the horizontal direction, one into each arm of the cross. The holes are placed so that both photons are trapped in the center where the arms cross. The blue and red curves represent the intensity of the electric fields of the respective photons. The photons interact due to the nonlinearity of the crystal that forms the cross. Credit: Eric Proctor
Army researchers predict quantum computer circuits that will no longer need extremely cold temperatures to function could become a reality after about a decade.
For years, solid-state quantum technology that operates at room temperature seemed remote. While the application of transparent crystals with optical nonlinearities had emerged as the most likely route to this milestone, the plausibility of such a system always remained in question.
Now, Army scientists have officially confirmed the validity of this approach. Dr. Kurt Jacobs, of the U.S. Army Combat Capabilities Development Command’s Army Research Laboratory, working alongside Dr. Mikkel Heuck and Prof. Dirk Englund, of the Massachusetts Institute of Technology, became the first to demonstrate the feasibility of a quantum logic gate comprised of photonic circuits and optical crystals.
“If future devices that use quantum technologies will require cooling to very cold temperatures, then this will make them expensive, bulky, and power hungry,” Heuck said. “Our research is aimed at developing future photonic circuits that will be able to manipulate the entanglement required for quantum devices at room temperature.”
Quantum technology offers a range of future advances in computing, communications, and remote sensing.
In order to accomplish any kind of task, traditional classical computers work with information that is fully determined. The information is stored in many bits, each of which can be on or off. A classical computer, when given an input specified by a number of bits, can process this input to produce an answer, which is also given as a number of bits. A classical computer processes one input at a time.
In contrast, quantum computers store information in qubits that can be in a strange state where they are both on and off at the same time. This allows a quantum computer to explore the answers to many inputs at the same time. While it cannot output all the answers at once, it can output relationships between these answers, which allows it to solve some problems much faster than a classical computer.
Unfortunately, one of the major drawbacks of quantum systems is the fragility of the strange states of the qubits. Most prospective hardware for quantum technology must be kept at extremely cold temperatures—close to zero kelvins—to prevent the special states being destroyed by interacting with the computer’s environment.
“Any interaction that a qubit has with anything else in its environment will start to distort its quantum state,” Jacobs said. “For example, if the environment is a gas of particles, then keeping it very cold keeps the gas molecules moving slowly, so they don’t crash into the quantum circuits as much.”
Researchers have directed various efforts to resolve this issue, but a definite solution is yet to be found. At the moment, photonic circuits that incorporate nonlinear optical crystals have presently emerged as the sole feasible route to quantum computing with solid-state systems at room temperatures.
“Photonic circuits are a bit like electrical circuits, except they manipulate light instead of electrical signals,” Englund said. “For example, we can make channels in a transparent material that photons will travel down, a bit like electrical signals traveling along wires.”
Unlike quantum systems that use ions or atoms to store information, quantum systems that use photons can bypass the cold temperature limitation. However, the photons must still interact with other photons to perform logic operations. This is where the nonlinear optical crystals come into play.
Researchers can engineer cavities in the crystals that temporarily trap photons inside. Through this method, the quantum system can establish two different possible states that a qubit can hold: a cavity with a photon (on) and a cavity without a photon (off). These qubits can then form quantum logic gates, which create the framework for the strange states.
In other words, researchers can use the indeterminate state of whether or not a photon is in a crystal cavity to represent a qubit. The logic gates act on two qubits together, and can create “quantum entanglement” between them. This entanglement is automatically generated in a quantum computer, and is required for quantum approaches to applications in sensing.
However, scientists based the idea to make quantum logic gates using nonlinear optical crystals entirely on speculation — up until this point. While it showed immense promise, doubts remained as to whether this method could even lead to practical logic gates.
The application of nonlinear optical crystals had remained in question until researchers at the Army’s lab and MIT presented a way to realize a quantum logic gate with this approach using established photonic circuit components.
“The problem was that if one has a photon travelling in a channel, the photon has a ‘wave-packet’ with a certain shape,” Jacobs said. “For a quantum gate, you need the photon wave-packets to remain the same after the operation of the gate. Since nonlinearities distort wave-packets, the question was whether you could load the wave-packet into cavities, have them interact via a nonlinearity, and then emit the photons again so that they have the same wave-packets as they started with.”
Once they designed the quantum logic gate, the researchers performed numerous computer simulations of the operation of the gate to demonstrate that it could, in theory, function appropriately. Actual construction of a quantum logic gate with this method will first require significant improvements in the quality of certain photonic components, researchers said.
“Based on the progress made over the last decade, we expect that it will take about ten years for the necessary improvements to be realized,” Heuck said. “However, the process of loading and emitting a wave-packet without distortion is something that we should able to realize with current experimental technology, and so that is an experiment that we will be working on next.”
Physical Review Letters published the team’s findings in a peer-reviewed paper on April 20, 2020.
Reference: “Controlled-Phase Gate Using Dynamically Coupled Cavities and Optical Nonlinearities” by Mikkel Heuck, Kurt Jacobs and Dirk R. Englund, 20 April 2020, Physical Review Letters.
DOI: 10.1103/PhysRevLett.124.160501
Thanks for sharing.
Just build the quantum computer cores in space, the outside temperature should be cold enough or use the existing or Elons’ Sat-trains! 🛰️
Set your mainframes adrift in the cool reaches of the free oceans of cool space!