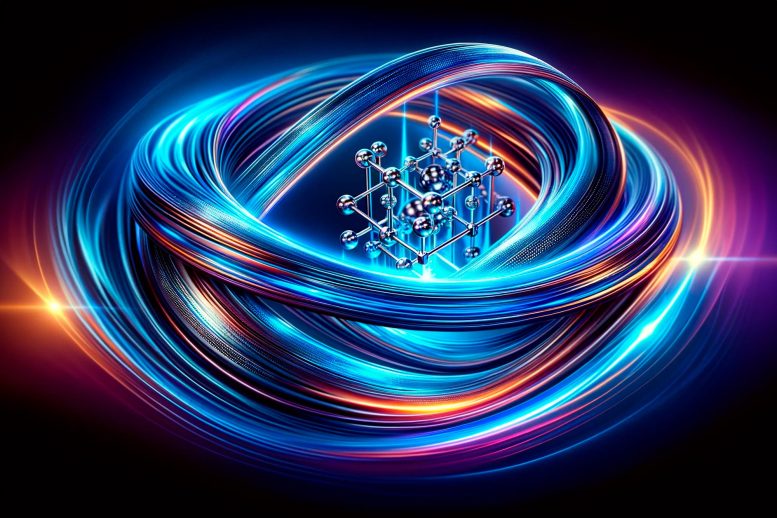
Rice University’s groundbreaking research on a “strange metal” quantum material reveals unconventional electrical flow, defying traditional quasiparticle theory. This discovery, made through detailed shot noise experiments, suggests a novel understanding of charge movement in strange metals and hints at a broader, universal phenomenon in quantum materials.
Rice physicists find evidence of exotic charge transport in quantum material.
True to form, a “strange metal” quantum material proved strangely quiet in recent quantum noise experiments at Rice University. Published on November 23 in the journal Science, the measurements of quantum charge fluctuations known as “shot noise” provide the first direct evidence that electricity seems to flow through strange metals in an unusual liquidlike form that cannot be readily explained in terms of quantized packets of charge known as quasiparticles.
“The noise is greatly suppressed compared to ordinary wires,” said Rice’s Douglas Natelson, the study’s corresponding author. “Maybe this is evidence that quasiparticles are not well-defined things or that they’re just not there and charge moves in more complicated ways. We have to find the right vocabulary to talk about how charge can move collectively.”
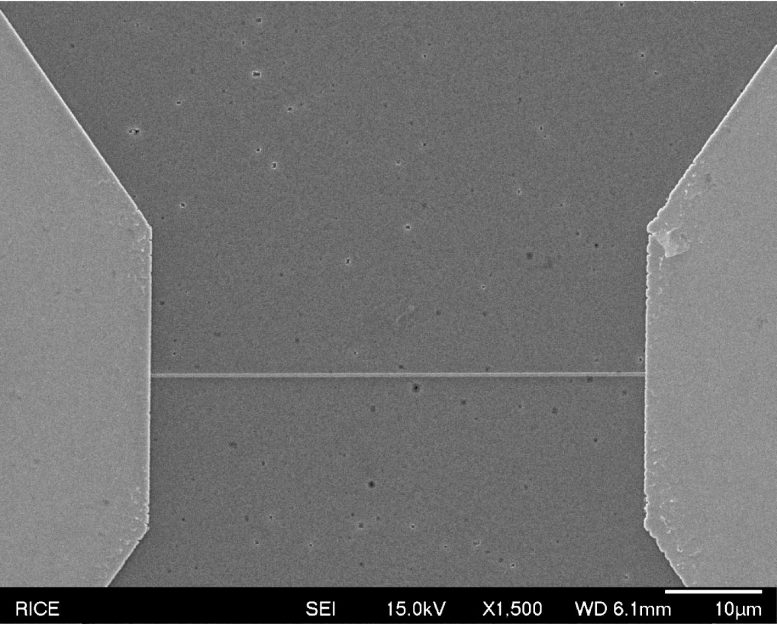
Scanning electron microscope image of a long YbRh2Si2 wire. Credit: Liyang Chen/Natelson research group/Rice University
Experiments on Quantum Critical Materials
The experiments were performed on nanoscale wires of a quantum critical material with a precise 1-2-2 ratio of ytterbium, rhodium, and silicon (YbRh2Si2), which has been studied in great depth during the past two decades by Silke Paschen, a solid-state physicist at the Vienna University of Technology (TU Wien). The material contains a high degree of quantum entanglement that produces a very unusual (“strange”) temperature-dependent behavior that is very different from the one in normal metals such as silver or gold.
In such normal metals, each quasiparticle, or discrete unit, of charge is the product of incalculable tiny interactions between countless electrons. First put forward 67 years ago, the quasiparticle is a concept physicists use to represent the combined effect of those interactions as a single quantum object for the purposes of quantum mechanical calculations.
Theory and Empirical Evidence
Some prior theoretical studies have suggested that the charge in a strange metal might not be carried by such quasiparticles, and shot noise experiments allowed Natelson, study lead author Liyang Chen, a former student in Natelson’s lab, and other Rice and TU Wien co-authors to gather the first direct empirical evidence to test the idea.
“The shot noise measurement is basically a way of seeing how granular the charge is as it goes through something,” Natelson said. “The idea is that if I’m driving a current, it consists of a bunch of discrete charge carriers. Those arrive at an average rate, but sometimes they happen to be closer together in time, and sometimes they’re farther apart.”
Technical Challenges and Breakthroughs
Applying the technique in YbRh2Si2 crystals presented significant technical challenges. Shot noise experiments cannot be performed on single macroscopic crystals but, rather, require samples of nanoscopic dimensions. Thus, the growth of extremely thin but nevertheless perfectly crystalline films had to be achieved, something that Paschen, Maxwell Andrews and their collaborators at TU Wien managed after almost a decade of hard work. Next, Chen had to find a way to maintain that level of perfection while fashioning wires from these thin films that were about 5,000 times narrower than a human hair.
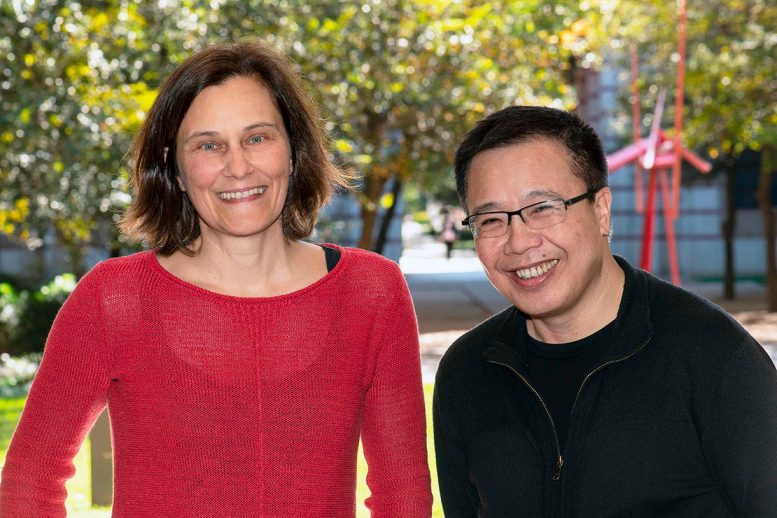
Physicists Silke Paschen (left) of Vienna University of Technology and Qimiao Si of Rice University. Credit: Tommy LaVergne/Rice University
Theoretical Perspectives and Future Implications
Rice co-author Qimiao Si, the lead theorist on the study and the Harry C. and Olga K. Wiess Professor of Physics and Astronomy, said he, Natelson and Paschen first discussed the idea for the experiments while Paschen was a visiting scholar at Rice in 2016. Si said the results are consistent with a theory of quantum criticality he published in 2001 that he has continued to explore in a nearly two-decade collaboration with Paschen.
“The low shot noise brought about fresh new insights into how the charge-current carriers entwine with the other agents of the quantum criticality that underlies the strange metallicity,” said Si, whose group performed calculations that ruled out the quasiparticle picture. “In this theory of quantum criticality, the electrons are pushed to the verge of localization, and the quasiparticles are lost everywhere on the Fermi surface.”
Natelson said the larger question is whether similar behavior might arise in any or all of the dozens of other compounds that exhibit strange metal behavior.
“Sometimes you kind of feel like nature is telling you something,” Natelson said. “This ‘strange metallicity’ shows up in many different physical systems, despite the fact that the microscopic, underlying physics is very different. In copper-oxide superconductors, for example, the microscopic physics is very, very different than in the heavy-fermion system we’re looking at. They all seem to have this linear-in-temperature resistivity that’s characteristic of strange metals, and you have to wonder if there is something generic going on that is independent of whatever the microscopic building blocks are inside them.”
Reference: “Shot noise in a strange metal” by Liyang Chen, Dale T. Lowder, Emine Bakali, Aaron Maxwell Andrews, Werner Schrenk, Monika Waas, Robert Svagera, Gaku Eguchi, Lukas Prochaska, Yiming Wang, Chandan Setty, Shouvik Sur, Qimiao Si, Silke Paschen and Douglas Natelson, 23 November 2023, Science.
DOI: 10.1126/science.abq6100
The research was supported by the Department of Energy’s Basic Energy Sciences program (DE-FG02-06ER46337), the National Science Foundation (1704264, 2220603), the European Research Council (101055088), the Austrian Science Fund (FWF I4047, FWF SFB F 86), the Austrian Research Promotion Agency (FFG 2156529, FFG 883941), the European Union’s Horizon 2020 program (824109-EMP), the Air Force Office of Scientific Research (FA8665-22-1-7170), the Welch Foundation (C-1411) and the Vannevar Bush Faculty Fellowship (ONR-VB-N00014-23-1-2870).
According to topological vortex gravitational field theory, there is no eternal mass, only eternal fluid mechanics.
If you are interested, you can browse the comments of https://scitechdaily.com/microscope-spacecrafts-most-precise-test-of-key-component-of-the-theory-of-general-relativity/.
Good luck to you all.
I see it as sharing or exchanging of electrons and when spin values reach equal value then there is no collision in moving the charge, no noise.
Please answer:
Where does spin come from? Why spin?
Theory needs to be updated.
Good luck to you.
Magnetic balls can pair up in clumps or run in long strings. If the string runs the length of the sample there’s not a lot of excuse for sample noise. It’s not that entanglements don’t explain the results, it’s the lack of clumping evident in long entanglement streams. Entanglement links don’t have to be tight spacings in low noise crystal wires. It’s also not so much that localized lattice deformations are critical, it’s that magnetic entanglements are naturally self-shielding extendable groupings. My 2 cents, anyway.
The “magnetic balls” there are supposed to represent electrons, of course. Side note: more generally they probably should be modelled as field generator ellipsoids, giving due respect to their poles. The effect of the superconductive lattice on a stream of electrons is that, due to a Meisnner-type of effect, both components of the mix have their magnetic fields mutually compressed in directions lateral to relative motion. Manipulation of the lateral magnetic fields can eliminate the energy/impact of the lateral electrostatic fields. Atoms of the superconductor are entangled together fully, electrons are thus compelled into entangling together and spinning as a unit independent of the superconductor by this.