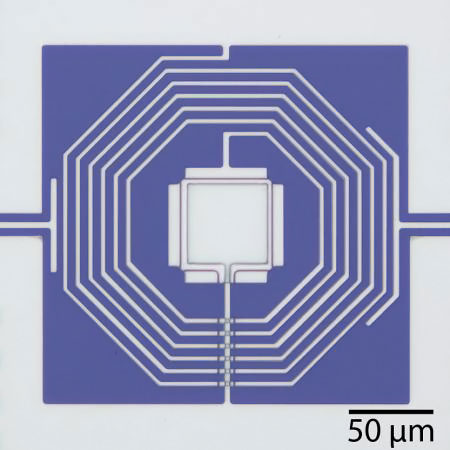
Caltech researchers achieve first-ever observation and control of quantum motion in a visible-sized object. Credit: Chan Lei and Keith Schwab/Caltech
For the first time, a team of physicists has discovered a way to observe and control quantum motion of an object that is large enough to see.
Consider the pendulum of a grandfather clock. If you forget to wind it, you will eventually find the pendulum at rest, unmoving. However, this simple observation is only valid at the level of classical physics—the laws and principles that appear to explain the physics of relatively large objects at human scale. However, quantum mechanics, the underlying physical rules that govern the fundamental behavior of matter and light at the atomic scale, state that nothing can quite be completely at rest.
For the first time, a team of Caltech researchers and collaborators has found a way to observe—and control—this quantum motion of an object that is large enough to see. Their results are published in the August 27 online issue of the journal Science.
Researchers have known for years that in classical physics, physical objects indeed can be motionless. Drop a ball into a bowl, and it will roll back and forth a few times. Eventually, however, this motion will be overcome by other forces (such as gravity and friction), and the ball will come to a stop at the bottom of the bowl.
“In the past couple of years, my group and a couple of other groups around the world have learned how to cool the motion of a small micrometer-scale object to produce this state at the bottom, or the quantum ground state,” says Keith Schwab, a Caltech professor of applied physics, who led the study. “But we know that even at the quantum ground state, at zero temperature, very small amplitude fluctuations—or noise—remain.”
Because this quantum motion, or noise, is theoretically an intrinsic part of the motion of all objects, Schwab and his colleagues designed a device that would allow them to observe this noise and then manipulate it.
The micrometer-scale device consists of a flexible aluminum plate that sits atop a silicon substrate. The plate is coupled to a superconducting electrical circuit as the plate vibrates at a rate of 3.5 million times per second. According to the laws of classical mechanics, the vibrating structures eventually will come to a complete rest if cooled to the ground state.
But that is not what Schwab and his colleagues observed when they actually cooled the spring to the ground state in their experiments. Instead, the residual energy—quantum noise—remained.
“This energy is part of the quantum description of nature—you just can’t get it out,” says Schwab. “We all know quantum mechanics explains precisely why electrons behave weirdly. Here, we’re applying quantum physics to something that is relatively big, a device that you can see under an optical microscope, and we’re seeing the quantum effects in a trillion atoms instead of just one.”
Because this noisy quantum motion is always present and cannot be removed, it places a fundamental limit on how precisely one can measure the position of an object.
But that limit, Schwab and his colleagues discovered, is not insurmountable. The researchers and collaborators developed a technique to manipulate the inherent quantum noise and found that it is possible to reduce it periodically. Coauthors Aashish Clerk from McGill University and Florian Marquardt from the Max Planck Institute for the Science of Light proposed a novel method to control the quantum noise, which was expected to reduce it periodically. This technique was then implemented on a micron-scale mechanical device in Schwab’s low-temperature laboratory at Caltech.
“There are two main variables that describe the noise or movement,” Schwab explains. “We showed that we can actually make the fluctuations of one of the variables smaller—at the expense of making the quantum fluctuations of the other variable larger. That is what’s called a quantum squeezed state; we squeezed the noise down in one place, but because of the squeezing, the noise has to squirt out in other places. But as long as those more noisy places aren’t where you’re obtaining a measurement, it doesn’t matter.”
The ability to control quantum noise could one day be used to improve the precision of very sensitive measurements, such as those obtained by LIGO, the Laser Interferometry Gravitational-wave Observatory, a Caltech-and-MIT-led project searching for signs of gravitational waves, ripples in the fabric of space-time.
“We’ve been thinking a lot about using these methods to detect gravitational waves from pulsars—incredibly dense stars that are the mass of our sun compressed into a 10 km radius and spin at 10 to 100 times a second,” Schwab says. “In the 1970s, Kip Thorne [Caltech’s Richard P. Feynman Professor of Theoretical Physics, Emeritus] and others wrote papers saying that these pulsars should be emitting gravity waves that are nearly perfectly periodic, so we’re thinking hard about how to use these techniques on a gram-scale object to reduce quantum noise in detectors, thus increasing the sensitivity to pick up on those gravity waves,” Schwab says.
In order to do that, the current device would have to be scaled up. “Our work aims to detect quantum mechanics at bigger and bigger scales, and one day, our hope is that this will eventually start touching on something as big as gravitational waves,” he says.
These results were published in an article titled, “Quantum squeezing of motion in a mechanical resonator.” In addition to Schwab, Clerk, and Marquardt, other coauthors include former graduate student Emma E. Wollman (PhD ’15); graduate students Chan U. Lei and Ari J. Weinstein; former postdoctoral scholar Junho Suh; and Andreas Kronwald of Friedrich-Alexander-Universität in Erlangen, Germany. The work was funded by the National Science Foundation (NSF), the Defense Advanced Research Projects Agency, and the Institute for Quantum Information and Matter, an NSF Physics Frontiers Center that also has support from the Gordon and Betty Moore Foundation.
Reference: “Quantum squeezing of motion in a mechanical resonator” by E. E. Wollman, C. U. Lei, A. J. Weinstein, J. Suh, A. Kronwald, F. Marquardt, A. A. Clerk and K. C. Schwab, 28 August 2015, Science.
DOI: 10.1126/science.aac5138
I wonder if this is related to one day developing interstellar travel?
No. There will NEVER be “interstellar” travel as in science fiction. The universe is too big and the limited speed of travel is not fast enough to get anywhere interesting in any several lifetimes.
Never say never…
Noise in the form of systematized mantras as repetitive interaction shift plane of electrons towards the center of atom by emitting radiation and towards inner orbit absorb energy of radiation by quantum absorption. Saint Thirumoolar acousto optic mantras doing a three plane interaction using 8/16 deviated algorithm.Hence noise filtering is essential.Using 16 square deviations of embedded copper plate acousto optic effect and further emission was controlled.
Sankaravelayudhan Nandakumar,Astrogeneticist
I do not see this as anything other than a peculiarity.
Since quantum noise is high frequency all you have to do is insert a more or less similar noise into the device. Eventually they will synchronize with opposite polarities and will reduce the noise in a very small area for a short period of time while increasing the noise everywhere else. But since quantum noise is unpredictable you can’t say when it will occur but only that it probably will.
Instead of “more or less similar noise”, wouldn’t you need to carefully inject noise of the same frequency and amplitude in order to get the cancelling you desire? Wouldn’t injecting unpredictable (random) noise more likely increase the total noise, and in some cases cause harmonics?
Apparently this quantum noise is not totally unpredictable, since these scientists are able to “squeeze” it.
I believe the most crucial part that would reveal a lot of mystery is where the “transition” between the quantum scale and the classical scale takes place.
Also, suppressing the quantum noise would help improve the sensitivity of Dark Matter detectors.
Quantum motion should be studied, it could be the basic energy that forms matter? Everything combined is one whole thing. We sometimes separate it in our erroneous imagination, but it is really all one thing. All is connected and united as the one and only Universe. The Universe is everywhere without beginning or end. Space is the infinite unity. Space is full of constant motion. The motion of space is eternally vibrating waves in all directions. All particles, people, planets, and galaxies are surrounded, formed, connected and powered by a unified background of energy/information waves constantly passing through all of space from infinity. The undetected immanent background of energy waves continually passes through us and the entire Universe. It all moves together. This perpetual wave motion is the basic energy behind all forces, life, time and matter. All matter is a temporary form of endless energy.
Infinite space is always moving, bending, pulsing, twisting, and spinning within itself. Waves of space, always have and always will, alternately compress and stretch areas of space. Waves pass through each other from all directions intersecting, forming spherical standing waves. Repeated wave motion results in matter, “spinning bits of space,” electrons, quarks, positrons, and all particles.
Tap a small shallow round plastic kid pool filled with water once on it’s edge. Watch waves head toward the center, form standing waves and continue through. Now imagine, instead of circular waves on a flat water surface, 3-D spherical waves of space. Experiments detecting particles appearing and disappearing in a total vacuum can prove that all of space has a background of wave energy that forms all matter. Thinking individual separate particles leads to confusion, get the big picture. It all moves together.
Maybe…
But it is easier to understand the big picture if you can understand the smaller parts that make up that picture.