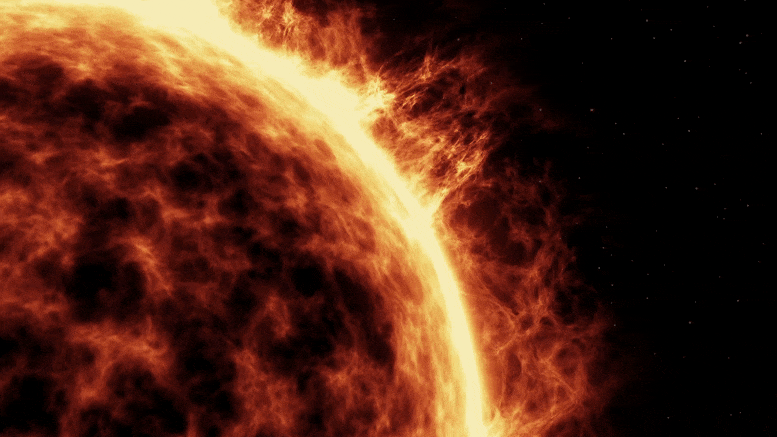
Astronomers have finally resolved the conflict between the internal structure of the Sun as determined from solar oscillations and the structure derived from the fundamental theory of stellar evolution.
New calculations of Solar spectrum resolve decade-long controversy about the composition of our star.
Although our sun is far closer than any other star in the universe, it still has its mysteries. After all, it is still 93 million miles (150 million kilometers) from Earth and we have just a narrow perspective. Plus its surface is blistering hot, its atmosphere even hotter, and it’s constantly blasting out particles at about 1 million miles per hour. It’s no wonder we’re still making new discoveries.
In fact, astronomers have just resolved the decade-long solar abundance crisis: the conflict between the internal structure of the Sun as determined from solar oscillations (helioseismology) and the structure derived from the fundamental theory of stellar evolution, which in turn relies on measurements of the present-day Sun’s chemical composition. New calculations of the physics of the Sun’s atmosphere yield updated results for abundances of different chemical elements, which resolve the conflict. Notably, the Sun contains more oxygen, silicon, and neon than previously thought. The methods employed also promise considerably more accurate estimates of the chemical compositions of stars in general.
What do you do when a tried-and-true method for determining the Sun’s chemical composition appears to be at odds with an innovative, precise technique for mapping the Sun’s inner structure? That was the situation facing astronomers studying the Sun until new calculations, now published by Ekaterina Magg, Maria Bergemann, and colleagues, resolved the apparent contradiction.
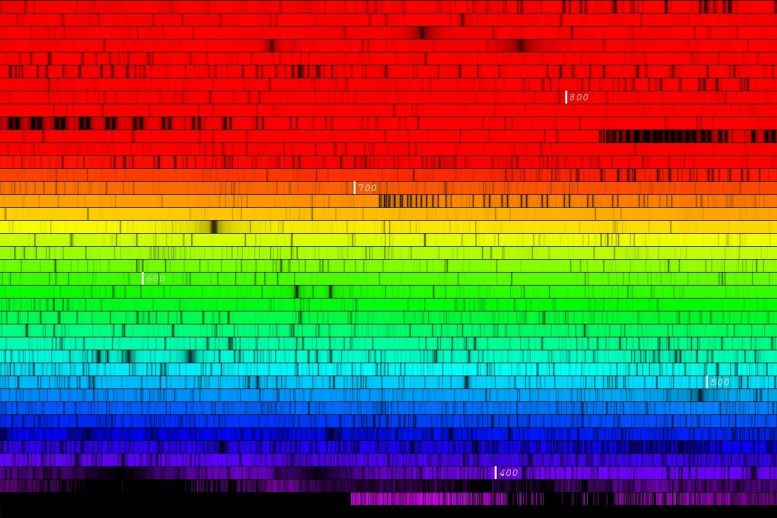
Spectrum of the Sun, taken with the NARVAL very high-resolution spectrograph installed at the Télescope Bernard Lyot, Observatoire Midi-Pyrénées. Spectra such as this, in particular the properties of the dark absorption lines that are clearly visible in this image, allow astronomers to deduce a star’s temperature and chemical composition. Credit: © M. Bergemann / MPIA / NARVAL@TBL
The tried-and-true method in question is spectral analysis. In order to determine the chemical composition of our Sun, or of any other star out there, astronomers routinely turn to spectra: the rainbow-like decomposition of light into its different wavelengths. Stellar spectra contain conspicuous, sharp dark lines, first noticed by William Wollaston in 1802, famously rediscovered by Joseph von Fraunhofer in 1814, and identified as tell-tale signs indicating the presence of specific chemical elements by Gustav Kirchhoff and Robert Bunsen in the 1860s.
Sun Facts
- Type: G-type main-sequence star (G2V)
- Informal Type: Yellow Dwarf
- Age: ~4.5 billion years
- Volume: 1.3 million times Earth’s
- Core Temperature: 27 million degrees Fahrenheit (15 million degrees Celsius)
- Distance From Earth: 93 million miles (150 million kilometers)
- Distance From Galactic Center: 26,000 light years
Pioneering work by the Indian astrophysicist Meghnad Saha in 1920 related the strength of those “absorption lines” to stellar temperature and chemical composition, providing the basis for our physical models of stars. Cecilia Payne-Gaposchkin’s realization that stars like our Sun consist mainly of hydrogen and helium, with no more than trace amounts of heavier chemical elements, is based on that work.
The underlying calculations relating spectral features to the chemical composition and physics of the stellar plasma have been of crucial importance to astrophysics ever since. They have been the foundation of a century-long progress in our understanding of the chemical evolution of the universe as well as of the physical structure and evolution of stars and exoplanets. That is why it came as something of a shock when, as new observational data became available and provided an insight into the inner workings of our Sun, the different pieces of the puzzle apparently did not fit together.
The modern standard model of solar evolution is calibrated using a famous (in solar physics circles) set of measurements of the solar atmosphere’s chemical composition, published in 2009. But in a number of important details, a reconstruction of our favorite star’s inner structure based on that standard model contradicts another set of measurements: helioseismic data, that is, measurements that track very precisely the minute oscillations of the Sun as a whole – the way that the Sun rhythmically expands and contracts in characteristic patterns, on time scales between seconds and hours.
Just like seismic waves provide geologists with crucial information about the Earth’s interior, or like the sound of a bell encodes information about its shape and material properties, helioseismology provides information about the interior of the Sun.
Highly accurate helioseismic measurements gave results about the Sun’s interior structure that were at odds with the solar standard models. According to helioseismology, the so-called convective region within our Sun where matter rises and sinks down again, like water in a boiling pot, was considerably larger than the standard model predicted. The speed of sound waves near the bottom of that region also deviated from the standard model’s predictions, as did the overall amount of helium in the Sun. To top it off, certain measurements of solar neutrinos – fleeting elementary particles, hard to detect, reaching us directly from the Sun’s core regions – were slightly off compared to experimental data, as well.
Astronomers had what they soon came to call a “solar abundances crisis,” and in search of a way out, some proposals ranged from the unusual to the downright exotic. Did the Sun maybe accrete some metal-poor gas during its planet-forming phase? Is energy being transported by the notoriously non-interacting dark matter particles?
The newly published study by Ekaterina Magg, Maria Bergemann, and colleagues has managed to resolve that crisis, by revisiting the models on which the spectral estimates of the Sun’s chemical composition are based. Early studies of how the spectra of stars are produced had relied on something known as local thermal equilibrium. They had assumed that locally, energy in each region of a star’s atmosphere has time to spread out and reach a kind of equilibrium. This would make it possible to assign to each such region a temperature, which leads to a considerable simplification in the calculations.
But astronomers had realized that this picture was oversimplified as early as the 1950s. Since then, more and more studies incorporated so-called Non-LTE calculations, dropping the assumption of local equilibrium. The Non-LTE calculations include a detailed description of how energy is exchanged within the system – atoms getting excited by photons, or colliding, photons getting emitted, absorbed or scattered. In stellar atmospheres, where densities are far too low to allow the system to reach thermal equilibrium, that kind of attention to detail pays off. There, Non-LTE calculations yield results that are markedly different from their local-equilibrium counterparts.
Maria Bergemann’s group at the Max Planck Institute for Astronomy is one of the world leaders when it comes to applying Non-LTE calculations to stellar atmospheres. As part of the work on her PhD in that group, Ekaterina Magg set out to calculate in more detail the interaction of radiation matter in the solar photosphere. The photosphere is the outer layer where most of the Sun’s light originates, and also where the absorption lines are imprinted on the solar spectrum.
In this study, they tracked all chemical elements that are relevant to the current models of how stars evolved over time, and applied multiple independent methods to describe the interactions between the Sun’s atoms and its radiation field in order to make sure their results were consistent. For describing the convective regions of our Sun, they used existing simulations that take into account both the motion of the plasma and the physics of radiation (“STAGGER” and “CO5BOLD”). For the comparison with spectral measurements, they chose the data set with the highest available quality: the solar spectrum published by the Institute for Astro- and Geophysics, University of Göttingen. “We also extensively focused on the analysis of statistical and systematic effects that could limit the accuracy of our results,” notes Magg.
The new calculations showed that the relationship between the abundances of these crucial chemical elements and the strength of the corresponding spectral lines was significantly different from what previous authors had claimed. Consequently, the chemical abundances that follow from the observed solar spectrum are somewhat different than those stated in the previous analysis.
“We found, that according to our analysis the Sun contains 26% more elements heavier than helium than previous studies had deduced,” explains Magg. In astronomy, such elements heavier than helium are called “metals.” Only on the order of a thousandth of a percent of all atomic nuclei in the Sun are metals; it is this very small number that has now changed by 26% of its previous value. Magg adds: “The value for the oxygen abundance was almost 15% higher than in previous studies.” The new values are, however, in good agreement with the chemical composition of primitive meteorites (“CI chondrites”) that are thought to represent the chemical makeup of the very early solar system.
When those new values are used as the input for current models of solar structure and evolution, the puzzling discrepancy between the results of those models and helioseismic measurements disappears. The in-depth analysis by Magg, Bergemann, and their colleagues of how spectral lines are produced, with its reliance on considerably more complete models of the underlying physics, manages to resolve the solar abundance crisis.
Maria Bergemann says: “The new solar models based on our new chemical composition are more realistic than ever before: they produce a model of the Sun that is consistent with all the information we have about the Sun’s present-day structure – sound waves, neutrinos, luminosity, and the Sun’s radius – without the need for non-standard, exotic physics in the solar interior.”
As an added bonus, the new models are easy to apply to stars other than the Sun. At a time where large-scale surveys like SDSS-V and 4MOST are providing high-quality spectra for an ever greater number of stars, this kind of progress is valuable indeed – putting future analyses of stellar chemistry, with their broader implications for reconstructions of the chemical evolution of our cosmos, on a firmer footing than ever before.
Reference: “Observational constraints on the origin of the elements: IV. Standard composition of the Sun” by Ekaterina Magg, Maria Bergemann, Aldo Serenelli, Manuel Bautista, Bertrand Plez, Ulrike Heiter, Jeffrey M. Gerber, Hans-Günter Ludwig, Sarbani Basu, Jason W. Ferguson, Helena Carvajal Gallego, Sébastien Gamrath, Patrick Palmeri and Pascal Quinet, 20 May 2022, Astronomy & Astrophysics.
DOI: 10.1051/0004-6361/202142971
Our universe, and the Sun as well, works much differently than the Lambda-CDM model suggests. Part of the Theory of Everything will describe exactly how the Sun created the “metals” it possesses to this day.
The core of our Sun is made of the ultimate energy of the universe. This energy uses the true strong force to exist and black holes are made purely of this plasma. Fusion is obviously not the ultimate energy which has been made obvious to science in the 80 years it has been created but never actually produced energy.
Our Sun began its life as a mass of what’s called quark plasma. This plasma uses the strong force between quarks and the particles that make up the extremely pressurized field of dark matter to exist. Dark matter is the ubiquitous catalyst instead of massive amounts of human-created electricity. Once quark plasma is created, it is the sheer density and pressure of dark matter that is able to keep the quarks apart indefinitely. The pressure of space actually overcomes the strong force and, as a result, space itself becomes the energy that is emitted from the mass as the strong force throws the incoming neutrinos out as energy. Black holes are optically invisible because they are absolute minimum entropy matter that only emit gamma rays. Dark matter is an extremely pressurized field of Tau neutrinos. The strong force is between quarks and these Tau neutrinos of space. Bosons break down into leptons which is what neutrinos are. Bosons were created to make the Big Bang theory allow a cloud of gas and dust to become a star.
Our Sun was a black hole and our planet and moon were as well. Of course, this sounds completely foreign to science but the solution to finally understand everything is naturally going to sound foreign just like when Galileo said the Earth was not the center of the universe.
Gravity did not create all the energy we see. A cloud of gas and dust is mistakenly responsible for creating all the forces that exist in science so that that cloud can become a star. This is not how the energy we see was created. Our universe turned itself into a gargantuan particle collider creating quark plasma shrapnel as the galaxies. The galaxies were at absolute minimum entropy the second they were formed in the collision as shrapnel. Each galaxy had its own size, shape, rotational rate, and trajectory. This plasma is optically invisible and can make shapes. Whether a galaxy was spiral, irregular, or elliptical was based on how much rotation the collision gave to the mass. Our galaxy was spinning.
Our galaxy used centrifugal force to create a disk with a bulbous center. The center separated from the disk and our black hole was formed. The remaining quark plasma disk was left to create all the solar systems that orbit the black hole to this day. The outer solar systems are going so fast because our galaxy was a single disk of plasma initially. That is the explanation for the problem that created the notion for dark matter in the first place.
Our solar system was a single mass of quark plasma as well and was spinning. Just like our galaxy, centrifugal force created a disk with a bulbous center. The center separated from the disk and our Sun was born as a black hole. The remaining quark plasma disk was left to create the planets and, just as everything before, the planets and their corresponding moons and rings were initially made of a single mass of quark plasma.
Now, to our Sun and its elements. Quark plasma creates all the naturally occurring elements all by itself from the outside of the mass inward. The Tau neutrinos of space finally start fusing with the quarks and create the first neutrons the mass will ever possess. This gives the black hole its first optical light since some resistance is now on the surface. The neutrons naturally break down to the first hydrogen the mass will possess. Then, the constantly forming neutrons fuse with the hydrogen to form the first helium atoms using the beta minus decay reaction. This is the only fusion our universe carries out. It is nothing but a method for the conservation of energy. That is why it has never produced a net-gain of energy and never will.
The beta minus decay reaction continues creating heavier elements on the surface of the quark plasma making the star darker until the light is extinguished and a surface forms. This is when the atmosphere is allowed to develop. That is what we live on. There is quark plasma at the core of our planet. How else would a 5 billion year old rock still leak magma from its surface with an energy source that is 4,000 miles away? Whatever is able to do that will be a plasma, not a solid.
The spectra of elements that we see are all on the surface of a quark star. Quark plasma is the limitless energy that provides the mass with the energy Sir Fred Hoyle stated needed to exist to create the heavier elements. It is quark plasma that science is looking for, not fusion. It has been proven fusion will never work for decades.
All the elements are on the surface of the star including iron. That is what sunspots are. Iron is a liquid at under 5,200°F and sunspots have measured at below 4,900°F. This means that pools of iron survive on the surface of the sun and even make indentions on the surface because of their weight.
I have just described the Theory of Everything. Of course, many do not want to hear it because they are under the false assumption that the Big Bang, Lambda-CDM model is a total fact. Anything else besides what has been taught for a century is “crackpot” even though the track record for current theories is less than zero. The Big Bang theory completely erases the importance of 95% of the mass of our universe which is what truly causes gravity. It makes science think fusion is, factually, the unlimited energy of the universe when it has been proven it isn’t.
The wait for the explanations for our universe is over. I have the whole story. I understand why Hubble did not want to make the universe expand and why Georges Lamaitre did. I understand that fork in the road was the biggest blunder science ever made. People that I converse with, especially professors and well educated people in astrophysics, do not want to hear anything about what I have to say because it isn’t what they have been told their when lives. Well, unfortunately, that is what paradigm shifts do to science. They completely upend everything that is known with a much more law-abiding, “Occam’s Razor” following explanation. There is no law that my theory doesn’t follow. That is why it is the Theory of Everything. It is the best explanation there is.