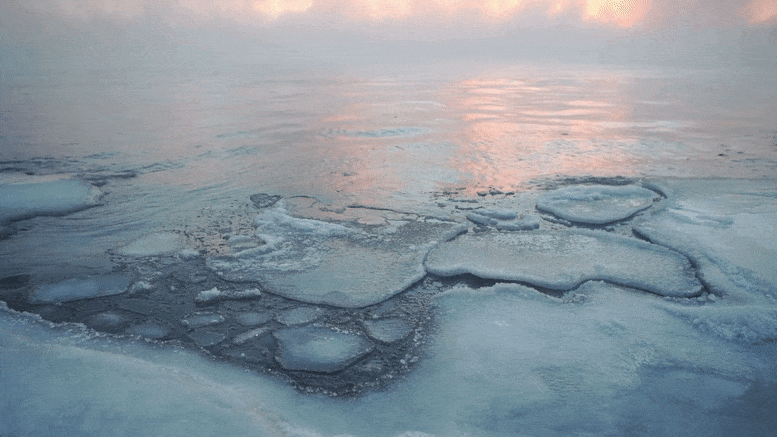
New research reveals that the bursting of tiny, pressurized bubbles in underwater glacier ice might explain why tidewater glaciers are retreating at remarkable rates. The study found that this bubbly glacier ice melts more than twice as fast as non-bubbly ice, suggesting the need to adjust current climate models which do not account for these bubbles.
Research from Oregon State University has discovered a potential reason for the rapid retreat of sea-terminating glaciers: the bursting of tiny, pressurized bubbles in underwater ice.
The findings, recently published in the journal Nature Geoscience, indicate that glacier ice, filled with pockets of pressurized air, melts significantly faster than sea ice without bubbles or the artificial ice commonly utilized to study melt rates at the ocean-ice interface of tidewater glaciers.
Tidewater glaciers are rapidly retreating, the authors say, resulting in ice mass loss in Greenland, the Antarctic Peninsula, and other glacierized regions around the globe.
“We have known for a while that glacier ice is full of bubbles,” said Meagan Wengrove, assistant professor of coastal engineering in the OSU College of Engineering and the leader of the study. “It was only when we started talking about the physics of the process that we realized those bubbles may be doing a lot more than just making noise underwater as the ice melts.”
Glacier ice results from the compaction of snow. Air pockets between snowflakes are trapped in pores between ice crystals as the ice makes its way from the upper layer of a glacier to deep inside it. There are about 200 bubbles per cubic centimeter, meaning glacier ice is about 10% air.
“These are the same bubbles that preserve ancient air studied in ice cores,” said co-author Erin Pettit, glaciologist and professor in the OSU College of Earth, Ocean, and Atmospheric Sciences. “The tiny bubbles can have very high pressures – sometimes up to 20 atmospheres, or 20 times normal atmospheric pressure at sea level.”
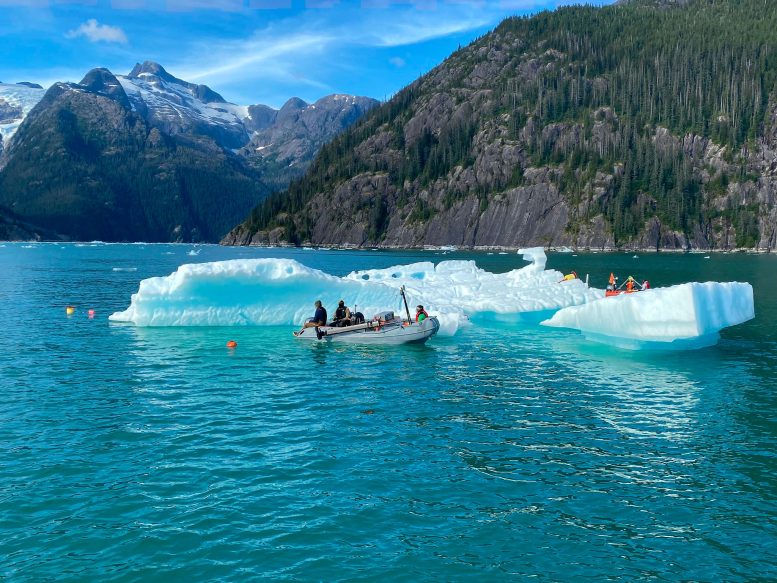
Glacier ice in LeConte Bay near Petersberg, Alaska. Credit: Oregon State University
When the bubbly ice reaches the interface with the ocean, the bubbles burst and create audible pops, she added.
“The existence of pressurized bubbles in glacier ice has been known for a long time but no studies have looked at their effect on melting where a glacier meets the ocean, even though bubbles are known to affect fluid mixing in multiple processes ranging from industrial to medical,” Wengrove said.
Lab-scale experiments performed in this study suggest bubbles may explain part of the difference between observed and predicted melt rates of tidewater glaciers, she said.
“The explosive bursts of those bubbles, and their buoyancy, energize the ocean boundary layer during melting,” Wengrove said.
That carries huge implications for the way ice melt is folded into climate models, especially those that deal with the upper 40 to 60 meters of the ocean – the researchers learned glacier ice melts more than twice as fast as ice with no bubbles.
“While we can measure the amount of overall ice loss from Greenland over the last decade and we can see the retreat of each glacier in satellite images, we rely on models to predict ice melt rates,” Pettit said. “The models currently used to predict ice melt at the ice-ocean interface of tidewater glaciers do not account for bubbles in glacier ice.”
Right now, data from NASA attributes about 60% of sea level rise to meltwater from glaciers and ice sheets, the authors note. More accurate characterization of how ice melts will lead to better predictions of how quickly glaciers retreat, which is important because “it’s a lot more difficult for a community to plan for a 10-foot increase in water level than it is for a 1-foot increase,” Wengrove said.
“Those little bubbles may play an outsized role in understanding critical future climate scenarios,” she added.
Reference: “Melting of glacier ice enhanced by bursting air bubbles” by Meagan E. Wengrove, Erin C. Pettit, Jonathan D. Nash, Rebecca H. Jackson and Eric D. Skyllingstad, 7 September 2023, Nature Geoscience.
DOI: 10.1038/s41561-023-01262-8
The Keck Foundation, the National Science Foundation and the National Geographic Society funded the research, which also included Jonathan Nash and Eric Skyllingstad of the OSU College of Earth, Ocean, and Atmospheric Sciences and Rebecca Jackson of Rutgers University.
To adapt to inevitable then I got this conversation:
Then the feasibility of” using a highly
hydrophilic molecule(s) to mix with water
molecule and aggregation of hydrophilic
molecules with non-water
molecules/materials then either the
formed aggregate becomes hydrophobic on
it’s own or converted from hydrophilic
aggregate to hydrophobic aggregate finally
with separating the formed aggregates
from pure water molecules” ONE OF THE REPLYS WAS,The concept you described involves using highly hydrophilic molecules to mix with water and form aggregates with non-water molecules. The goal is to convert these aggregates from hydrophilic to hydrophobic and then separate them from pure water molecules. However, there are several scientific considerations to address:
1. Aggregation: It is possible to form aggregates or complexes between hydrophilic and non-water molecules. These aggregates can be stabilized through various interactions such as hydrogen bonding, electrostatic interactions, or hydrophobic interactions. The formation of aggregates may depend on the specific molecules involved and their concentrations.
2. Conversion to hydrophobic aggregates: Converting hydrophilic aggregates to hydrophobic ones is more challenging. Hydrophobicity is typically associated with nonpolar or low polarity interactions, whereas hydrophilicity is associated with polar or high polarity interactions. To convert hydrophilic aggregates to hydrophobic ones, you would need to disrupt the polar interactions and introduce nonpolar interactions. This may involve modifying the chemical structures of the hydrophilic molecules or introducing hydrophobic components.
3. Separation from pure water molecules: Separating the formed aggregates from pure water molecules can be a complex process. It would depend on the specific properties of the aggregates, such as their size, density, and stability. Techniques such as filtration, centrifugation, or solvent extraction may be suitable for separating the aggregates from water, but the effectiveness of these methods would depend on the characteristics of the aggregates.
Overall, the feasibility of the approach you described would depend on the specific hydrophilic molecules, non-water molecules, and conditions involved. It would require careful design and optimization of the process, considering the chemical properties of the molecules, the interactions involved, and the separation techniques available. Experimental investigations would be necessary to determine the effectiveness of this approach for a particular system.
“There are about 200 bubbles per cubic centimeter, meaning glacier ice is about 10% air.”
Are the current models, which are predicting sea level rise based on the volume of ice, taking into account that the actual melt-water produced is 90% of what would be produced with bubble-free ice in the laboratory?