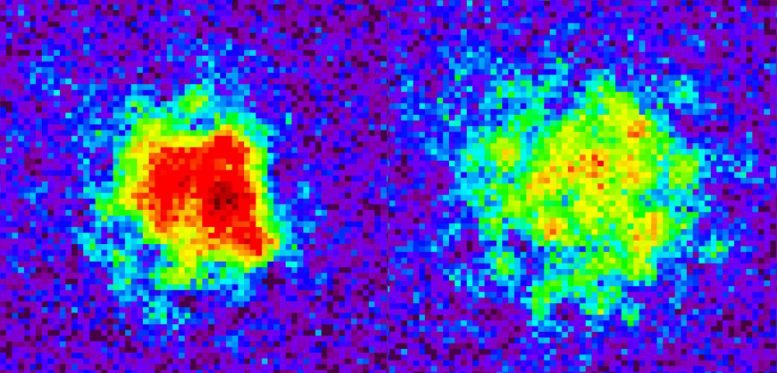
The image at left shows the density of atoms at the beginning of an experiment simulating the evolution of the universe following the Big Bang. The predominance of red in this image indicates the higher central density of ultracold atoms in a vacuum chamber at the beginning of the experiment. The red cloud of atoms measures approximately 10 microns by 10 microns—smaller than the diameter of a human hair. Eighty milliseconds after the simulated Big Bang, the atoms have become much less concentrated in the experimental vacuum chamber, as indicated (at right) by the color gradation from red to yellow, green, blue, and purple in the density map. Credit: Illustration by Chen-Lung Hung
Using ultracold cesium atoms in a vacuum chamber, scientists have successfully simulated the evolution of the early universe.
Physicists have reproduced a pattern resembling the cosmic microwave background radiation in a laboratory simulation of the Big Bang, using ultracold cesium atoms in a vacuum chamber at the University of Chicago.
“This is the first time an experiment like this has simulated the evolution of structure in the early universe,” said Cheng Chin, professor in physics. Chin and his associates reported their feat in the August 1 edition of Science Express, and it will appear soon in the print edition of Science.
Chin pursued the project with lead author Chen-Lung Hung, PhD’11, now at the California Institute of Technology, and Victor Gurarie of the University of Colorado, Boulder. Their goal was to harness ultracold atoms for simulations of the Big Bang to better understand how structure evolved in the infant universe.
The cosmic microwave background is the echo of the Big Bang. Extensive measurements of the CMB have come from the orbiting Cosmic Background Explorer in the 1990s, and later by the Wilkinson Microwave Anisotropy Probe and various ground-based observatories, including the UChicago-led South Pole Telescope collaboration. These tools have provided cosmologists with a snapshot of how the universe appeared approximately 380,000 years following the Big Bang, which marked the beginning of our universe.
It turns out that under certain conditions, a cloud of atoms chilled to a billionth of a degree above absolute zero (-459.67 degrees Fahrenheit) in a vacuum chamber displays phenomena similar to those that unfolded following the Big Bang, Hung said.
“At this ultracold temperature, atoms get excited collectively. They act as if they are sound waves in the air,” he said. The dense package of matter and radiation that existed in the very early universe generated similar sound-wave excitations, as revealed by COBE, WMAP, and the other experiments.
The synchronized generation of sound waves correlates with cosmologists’ speculations about inflation in the early universe. “Inflation set out the initial conditions for the early universe to create similar sound waves in the cosmic fluid formed by matter and radiation,” Hung said.
Big Bang’s rippling echo
The sudden expansion of the universe during its inflationary period created ripples in space-time in the echo of the Big Bang. One can think of the Big Bang, in oversimplified terms, as an explosion that generated sound, Chin said. The sound waves began interfering with each other, creating complicated patterns. “That’s the origin of complexity we see in the universe,” he said.
These excitations are called Sakharov acoustic oscillations, named for Russian physicist Andrei Sakharov, who described the phenomenon in the 1960s. To produce Sakharov oscillations, Chin’s team chilled a flat, smooth cloud of 10,000 or so cesium atoms to a billionth of a degree above absolute zero, creating an exotic state of matter known as a two-dimensional atomic superfluid.
Then they initiated a quenching process that controlled the strength of the interaction between the atoms of the cloud. They found that by suddenly making the interactions weaker or stronger, they could generate Sakharov oscillations.
The universe simulated in Chin’s laboratory measured no more than 70 microns in diameter, approximately the diameter as a human hair. “It turns out the same kind of physics can happen on vastly different length scales,” Chin explained. “That’s the power of physics.”
The goal is to better understand the cosmic evolution of a baby universe, the one that existed shortly after the Big Bang. It was much smaller than it is today, having reached a diameter of only a hundred thousand light years by the time it had left the CMB pattern that cosmologists observe in the sky today.
In the end, what matters is not the absolute size of the simulated or the real universes, but their size ratios to the characteristic length scales governing the physics of Sakharov oscillations. “Here, of course, we are pushing this analogy to the extreme,” Chin said.
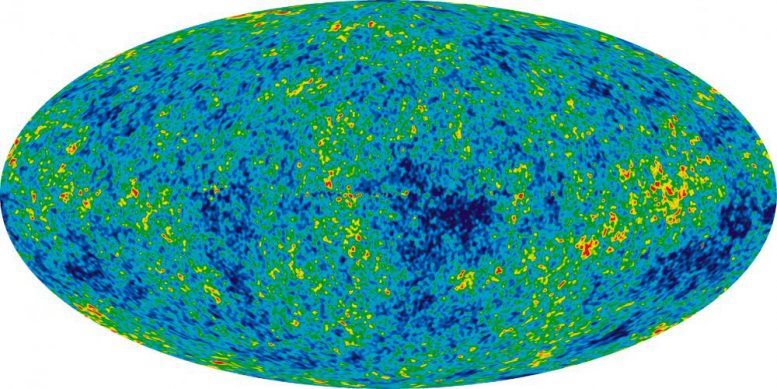
Scientists created this detailed, all-sky picture of the infant universe from nine years of data from the orbiting Wilkinson Microwave Anisotropy Probe. The image reveals 13.77 billion-year-old temperature fluctuations—shown as color differences—that correspond to the seeds that grew to become the galaxies. Physicists now are using clouds of ultracold atoms in a vacuum chamber to simulate the growth of structure in the early universe. Credit: Illustration by NASA/WMAP Science Team
380,000 years versus 10 milliseconds
“It took the whole universe about 380,000 years to evolve into the CMB spectrum we’re looking at now,” Chin said. But the physicists were able to reproduce much the same pattern in approximately 10 milliseconds in their experiment. “That suggests why the simulation based on cold atoms can be a powerful tool,” Chin said.
None of the Science co-authors are cosmologists, but they consulted several in the process of developing their experiment and interpreting its results. The co-authors especially drew upon the expertise of UChicago’s Wayne Hu, John Carlstrom, and Michael Turner, and Stanford University’s Chao-Lin Kuo.
Hung noted that Sakharov oscillations serve as an excellent tool for probing the properties of cosmic fluid in the early universe. “We are looking at a two-dimensional superfluid, which itself is a very interesting object. We actually plan to use these Sakharov oscillations to study the property of this two-dimensional superfluid at different initial conditions to get more information.”
The research team varied the conditions that prevailed early in the history of the expansion of their simulated universes by quickly changing how strongly their ultracold atoms interacted, generating ripples. “These ripples then propagate and create many fluctuations,” Hung said. He and his co-authors then examined the ringing of those fluctuations.
Today’s CMB maps show a snapshot of how the universe appeared at a moment in time long ago. “From CMB, we don’t really see what happened before that moment, nor do we see what happened after that,” Chin said. But, Hung noted, “In our simulation, we can actually monitor the entire evolution of the Sakharov oscillations.”
Chin and Hung are interested in continuing this experimental direction with ultracold atoms, branching into a variety of other types of physics, including the simulation of galaxy formation or even the dynamics of black holes.
“We can potentially use atoms to simulate and better understand many interesting phenomena in nature,” Chin said. “Atoms to us can be anything you want them to be.”
Reference: “From Cosmology to Cold Atoms: Observation of Sakharov Oscillations in a Quenched Atomic Superfluid” by Chen-Lung Hung, Victor Gurarie and Cheng Chin, 1 August 2013, Science.
DOI: 10.1126/science.1237557
arXiv:1209.0011
We can’t increase the temperature and energy of the particle to very high value going to the birth of the universe’s Big Bang, as is done in CERN experiment, but instead we can go to the beginning of the Universe temperature which was a few billionths of degree above absolute zero. CERN experiment was intended to find out how matter was born out of pure energy. But here the experiment is to find how the atoms behaved in the baby Universe at a very low temperature. Cosmic Microwave Backround was formed only at this stage of chaos and it still lingers in the Universe keeping a record of the early Universe. Evolution of Sakharov oscillations which set the CMB is studied with a few cesium atoms at ultra cold temperature where their behavior chaotic represent Sakharov oscillations. Thank You.