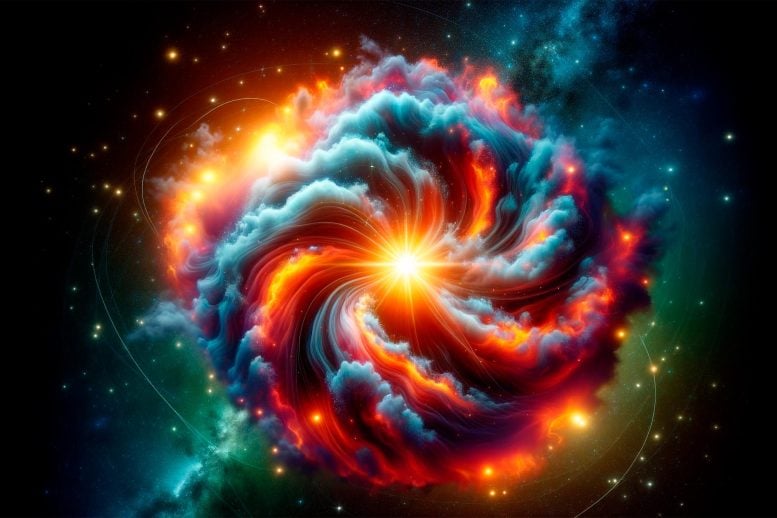
Researchers have confirmed a key aspect of star formation — the role of accretion disks and disk winds in overcoming the angular momentum problem. Using advanced radio telescope arrays, they observed the dynamics of disk winds in protostar systems, proving that these winds are crucial in facilitating star formation by mitigating rotational forces. Credit: SciTechDaily.com
New observations have confirmed a key step in the process of star formation: a rotating “cosmic wind” made of molecules, which is vitally important for collapsing gas clouds to contract sufficiently so as to form a hot, dense young star. The result was obtained from radio observations, combined with a sophisticated analysis that allowed astronomers to probe the flow of matter around a young star in the dark cloud CB26 in greater detail than ever before. The work has been published in the journal Astronomy & Astrophysics.
Observations undertaken by Ralf Launhardt, a group leader at the Max Planck Institute for Astronomy, and colleagues have clinched an important part of the standard scenario for the formation of new stars: a mechanism for how gas clouds can collapse to give birth to a new star, without being torn apart by their own rotation in the process.
New stars form when gas in a cosmic hydrogen cloud collapses under its own gravity, and its temperature rises. Beyond a certain threshold of density and temperature, nuclear fusion sets in, with hydrogen nuclei fusing to form helium nuclei. The energy that is set free by this process is what makes stars shine. But there is a complication. No gas cloud in the cosmos is perfectly still – all clouds rotate at least a bit. When the gas contracts, that rotation becomes ever faster. Physicists call this “angular momentum conservation.” Outside of astronomy, it is known from figure skating: When a figure skater wants to spin very fast, they begin a slow rotation with both arms and one leg stretched out. Then, they pull their limbs close to their axis of rotation, and the rotation speed of increases considerably.
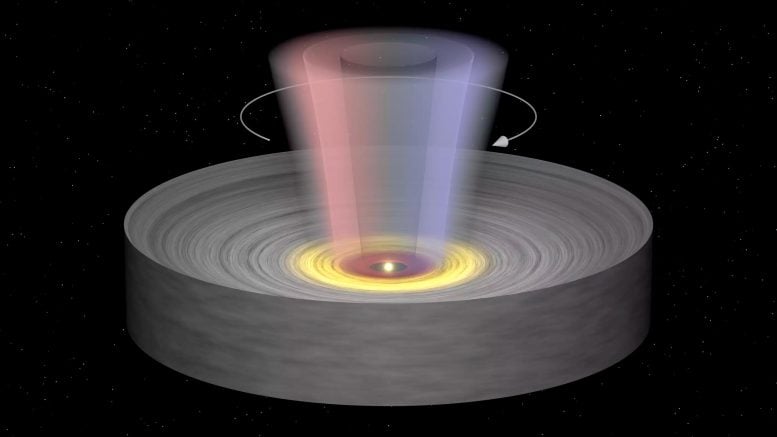
Artist’s impression of the geometry of the accretion disk around the young star and the outflowing rotating disk wind. Regions of the disk wind that are moving toward us appear blue-shifted and are hence colored in blue in the image; regions moving away from us are red-shifted (colored in red). Credit: © T. Müller, R. Launhardt (MPIA)
A Problem and Its (Potential) Solution
For star formation, this spells a potential problem. Fast spinning entails centrifugal forces, flinging matter away from the axis of rotation. For a swing ride or swing carousel, that is part of the fun: As the carousel rotates, the riders’ chain-supported chairs are flung outward. For a protostar, on the other hand, centrifugal forces could be fatal: If enough material is flung out as the cloud collapses and speeds up its spin, there might not be enough left to form a protostar in the first place!
This is known as the “angular momentum problem” of star formation. A theoretical solution for at least a large part of the problem was found in the 1980s. As additional matter falls onto the nascent central protostar, it forms a so-called accretion disk: a flat rotating disk of gas and dust, whose matter will eventually end up falling onto the protostar in the center. The physics behind accretion disks is rather involved: Some of the gas in the disk becomes a plasma, with hydrogen atoms separating into one electron and one proton each.
As the plasma is spun around in the disk, it creates a magnetic field. This field in turn influences the plasma flow: a small amount of plasma drifts off along the magnetic field lines. Ever so often, the drifting plasma particles collide with (electrically neutral) molecules; the result is that some of the molecular gas is carried away as well. These molecules make up a “disk wind,” which can take considerable angular momentum away from the disk. Loss of angular momentum slows the rotation, lessens the centrifugal forces, and could solve the protostar’s angular momentum problem.
From Hypothesis to Observation
At first, this scenario was no more than a plausible hypothesis. For an observer on Earth, a structure like an accretion disk around even the nearest newly-forming star is very small indeed. That is why it took more than 20 years for astronomers to find tentative evidence for rotation in this kind of mass flow: In 2009, Ralf Launhardt and colleagues at the Max Planck Institute for Astronomy were able to observe the outflow around a young star in a small hydrogen cloud with the designation CB26. With a distance of less than 460 light-years from Earth, CB26 is one of the closest known disk systems around a protostar.
The observations in question are made with radio telescopes operating at millimeter wavelengths, in this case an array of antennas called the Plateau de Bure Interferometer. In effect, such antennas are combined in a clever way so as to make them act like a single, much larger radio dish. Radio telescopes of this kind can detect radiation that is characteristic for different kinds of molecules – in this case, carbon monoxide (CO). When molecules move towards or away from the observer, that characteristic radiation is shifted to slightly longer or shorter wavelengths (“Doppler shift”), which in turn allows the astronomers to track the gas motion along the line of sight.
The 2009 observations showed that the gas outflow from the young star was indeed in motion, and in the just the right way one would expect from a rotating disk wind removing angular momentum. But they could not provide sufficiently fine details to allow any judgement about the distance from the star at which the wind was launched from the disk – a key property (think “leverage”) that determines how much angular momentum the gas flow can carry away.
Observing Rotating Disk Winds
The new results that have now been published clinch the case. For this work, Launhardt and colleagues were able to carry out observations with much higher angular resolution. They used a configuration of the Plateau de Bure observatory in which the radio antennas were placed much farther apart than in their first observations. They also fielded a sophisticated physico-chemical model of the disk, which allowed them to distinguish contributions from the disk and contributions from the disk wind.
All of this allowed the astronomers to pinpoint the dimensions of the cone-shaped outflow: near the disk, the lower end of the cone has a radius of roughly 1.5 times the Earth-Neptune distance – more than enough for the disk wind to carry away lots of angular momentum! This was the first time those dimensions had been determined directly from (reconstructed) images.
With these measurements, the argument was clinched: disk winds can indeed solve most of the angular momentum problem for protostars. Launhardt and colleagues were also able to compare their measurement with indirect reconstructions of disk wind dimensions, in nine other young star-disk systems that had been published since the 2009 paper. The comparison shows a clear trend for the average radius of the area in which the disk wind originates on the disk having grown over time: at the beginning, during the first tens of thousands of years, there are highly concentrated disk winds, while after about a million years the disk winds are much more diffuse.
Next Steps
The astronomers are already planning their next observations of CB26. In the meantime, the Plateau de Bure Interferometer has been upgraded. The new observatory, which is called NOEMA, has 12 antennas instead of the previous 6, and it provides for configurations that can tease out details twice as small as its predecessor could. But while those refinements hold considerable promise, the key step is the one taken in the present article: solid confirmation that disk winds are indeed a major factor in allowing protostars to form in the first place, and in solving the angular momentum problem.
Reference: “A resolved rotating disk wind from a young T Tauri star in the Bok globule CB 26” by R. Launhardt, Ya. N. Pavlyuchenkov, V. V. Akimkin, A. Dutrey, F. Gueth, S. Guilloteau, Th. Henning, V. Piétu, K. Schreyer, D. Semenov, B. Stecklum and T. L. Bourke, 17 October 2023, Astronomy & Astrophysics.
DOI: 10.1051/0004-6361/202347483
The MPIA researchers involved are Ralf Launhardt, Thomas Henning, and Dimitry Semenov in collaboration with Yaroslav Pavlyuchenkov, Vitaly Akimkin (both INASAN Moscow) and seven other scientists from Germany, France, and the UK.
Be the first to comment on "From Dust to Dazzle: Solving the Star-Making Puzzle"