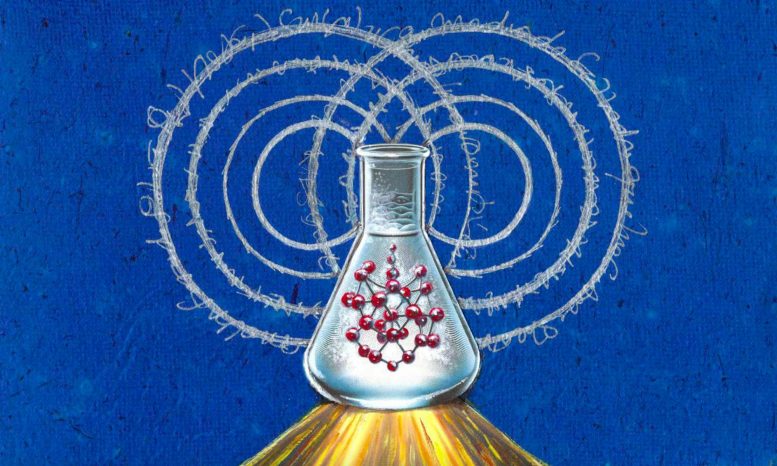
Rochester researchers have reported a strategy to understand how quantum coherence is lost for molecules in solvent with full chemical complexity. The findings open the door to the rational modulation of quantum coherence via chemical design and functionalization. Credit: Anny Ostau De Lafont
The findings can be used to design molecules with custom quantum coherence properties, laying the chemical foundation for emerging quantum technologies.
In quantum mechanics, particles can exist in multiple states at the same time, defying the logic of everyday experiences. This property, known as quantum superposition, is the basis for emerging quantum technologies that promise to transform computing, communication, and sensing. But quantum superpositions face a significant challenge: quantum decoherence. During this process, the delicate superposition of quantum states breaks down when interacting with its surrounding environment.
The Challenge of Quantum Decoherence
To unlock the power of chemistry to build complex molecular architectures for practical quantum applications, scientists need to understand and control quantum decoherence so that they can design molecules with specific quantum coherence properties. Doing so requires knowing how to rationally modify a molecule’s chemical structure to modulate or mitigate quantum decoherence. To that end, scientists need to know the “spectral density,” the quantity that summarizes how fast the environment moves and how strongly it interacts with the quantum system.
Breakthrough in Spectral Density Measurement
Until now, quantifying this spectral density in a way that accurately reflects the intricacies of molecules has remained elusive to theory and experimentation. But a team of scientists has developed a method to extract the spectral density for molecules in solvent using simple resonance Raman experiments—a method that captures the full complexity of chemical environments. Led by Ignacio Franco, an associate professor of chemistry and of physics at the University of Rochester, the team published their findings in the Proceedings of the National Academy of Sciences.
Linking Molecular Structure to Quantum Decoherence
Using the extracted spectral density, it is possible not only to understand how fast the decoherence happens but also to determine which part of the chemical environment is mostly responsible for it. As a result, scientists can now map decoherence pathways to connect molecular structure with quantum decoherence.
“Chemistry builds up from the idea that molecular structure determines the chemical and physical properties of matter. This principle guides the modern design of molecules for medicine, agriculture, and energy applications. Using this strategy, we can finally start to develop chemical design principles for emerging quantum technologies,” says Ignacio Gustin, a chemistry graduate student at Rochester and the first author of the study.
Resonance Raman Experiments: A Key Tool
The breakthrough came when the team recognized that resonance Raman experiments yielded all the information needed to study decoherence with full chemical complexity. Such experiments are routinely used to investigate photophysics and photochemistry, but their utility for quantum decoherence had not been appreciated. The key insights emerged from discussions with David McCamant, an associate professor in the chemistry department at Rochester and an expert in Raman spectroscopy, and with Chang Woo Kim, now on the faculty at Chonnam National University in Korea and an expert in quantum decoherence, while he was a postdoctoral researcher at Rochester.
Case Study: Thymine Decoherence
The team used their method to show, for the first time, how electronic superpositions in thymine, one of the building blocks of DNA, unravel in just 30 femtoseconds (one femtosecond is one-millionth of one billionth of a second) following its absorption of UV light. They found that a few vibrations in the molecule dominate the initial steps in the decoherence process, while solvent dominates the later stages. In addition, they discovered that chemical modifications to thymine can significantly alter the decoherence rate, with hydrogen-bond interactions near the thymine ring leading to more rapid decoherence.
Future Implications and Applications
Ultimately, the team’s research opens the way toward understanding the chemical principles that govern quantum decoherence. “We are excited to use this strategy to finally understand quantum decoherence in molecules with full chemical complexity and use it to develop molecules with robust coherence properties,” says Franco.
Reference: “Mapping electronic decoherence pathways in molecules” by Ignacio Gustin, Chang Woo Kim, David W. McCamant and Ignacio Franco, 28 November 2023, Proceedings of the National Academy of Sciences.
DOI: 10.1073/pnas.2309987120
In quantum mechanics, particles can exist in multiple states at the same time, defying the logic of everyday experiences. This statement is unscientific.
According to topological vortex gravitational field theory, not only particles, but almost all matter can exist in multiple states at the same time. Thismultiple states are reflected in the interaction of matter, including the observation and measurement of matter, and is also a form of material interaction.
For example, observing both the front and back of a topological vortex simultaneously, if the front is left-handed, then the back must be right-handed. The superposition of left-handed and right-handed states is like this to the observer, and the same applies to the interaction of vortices. The synchronous effect of the superposition and deflection of topological vortices makes the “one body with multiple states” more complex.
From accretion disks to quantum spins, topological vortices can provide infinite rotational force for many galaxies, celestial bodies, and microparticles.
The so-called quantum, its physical essence is the spin of topological vortices. Spin generates gravity. Spin generates energy. Spin generates evolution. Spin generation time.
Both academic journals and scientific workers must respect the seriousness of science. Scientists should not believe in pseudoscience like the Physical Review Letters (PRL) do. They firmly believe that two objects (such as two sets of cobalt-60) rotating in opposite directions can form a mirror image of each other.
The correct understanding and application of the rotation of topological vortices is an urgent issue in human science.
If anyone is really interested in science, you can browse https://www.zhihu.com/column/c_1278787135349633024.
Panchtatva is five state of material world solid,liquid, gas.
Forth state is plasma
Fifth state is panchtatva, is qutntam state Examples are heat wave sound wave ,Light wave which is generated from collision or striking effect of prton and electron or Electron to electron
“They found that a few vibrations in the molecule dominate the initial steps in the decoherence process, while solvent dominates the later stages. In addition, they discovered that chemical modifications to thymine can significantly alter the decoherence rate, with hydrogen-bond interactions near the thymine ring leading to more rapid decoherence.”
This doesn’t seem to resolve to molecules individually, but to group effects.
Beyond that, if people want to say the left side of an atom is in one place and the right side is in another place, no one should see a problem with a field description for that, but one atom centered on two places at the same time is perfectly unreal.
Quantum gravitometers rely on path differences between pairs of previously-entangled atoms, but some people try to sell the device as splitting a single atom in two, or they want to claim something strange is going on oddly quickly between distant locations.
As far as electrons in molecules or atoms go, they can jump quite quickly between states and they can probabilistically appear spread out widely over an atom or molecule, but again results in this case do not appear focused on a lone molecule and superposition statistics are necessarily built over plural detection cases, in any case. That is not to say electron superposition effects cannot possibly manifest directly or instantaneously, only that I’m not aware of a convincing example.
“I’m not aware of a convincing example.”
A hydrogen atom apparently doesn’t wobble as one might expect, due to its single electron behaving as if it is an enveloping shell, as if opposite sides of the electron are on opposite sides of the atom. Again, it all seems to make the most sense using a quantum field description, which inherently has atom-level centralized “nonlocalism” replacing a purely pointlike model.
“as if opposite sides of the electron are on opposite sides of the atom”
The geometry in that is so spin-2 as a composite of spin one components (paired field vectors) vs. spin-1/2. In that sense it also appears to be gravity-balanced while conserving a minimum bound EM energy, which is also impossible for me to miss here.
The difference is between an electron hidden within a balanced quantum orbital (a closed structure, or symmetry manifold, expressing 2 degrees of bound electron spin freedom, carried as if concealed within discrete event horizons as fully entangled half-fields), versus a partly disentangled exposed electron circling twice around an atom to either refuse passing photons or momentarily delay them. Existence of entangled half-fields implies instantaneous impacts on opposite sides of an atom (or super-atom) at an extreme, or some degree of gravity-based acceleration to critical speeds impacting not just electron effects but also light energy geometry itself in interfacing with orbital boundaries.
“… they discovered that chemical modifications to thymine can significantly alter the decoherence rate, with hydrogen-bond interactions near the thymine ring leading to more rapid decoherence.”
One of phizzorg’s premiere jackasses is bleating that the sublime vagueness in all of that is bad news for using bonded hydrogen to enhance cold entanglements between molecules or sheets of graphene. Figures. It’s actually a red flag. The anglo-gang there got all worked up a couple years ago when US mil patented a pressure-enabled high-temp superconductor, claimed it was impossible. One of the many memory-holed facets of the britwit gravity klan.
“hydrogen-bond interactions near the thymine ring leading to more rapid decoherence”
Hydrogen is critical to coherence/entanglement is all this press release is telling me. I mean “interactions” with hydrogen where? Hydrogen on noisy ambient reactant molecules or reactor impellor surfaces? One problem with such suggestions is they make some sense while killing the precious ambiguity. Maybe I should go back to being an intellectual property forensic examiner, so restless these days.