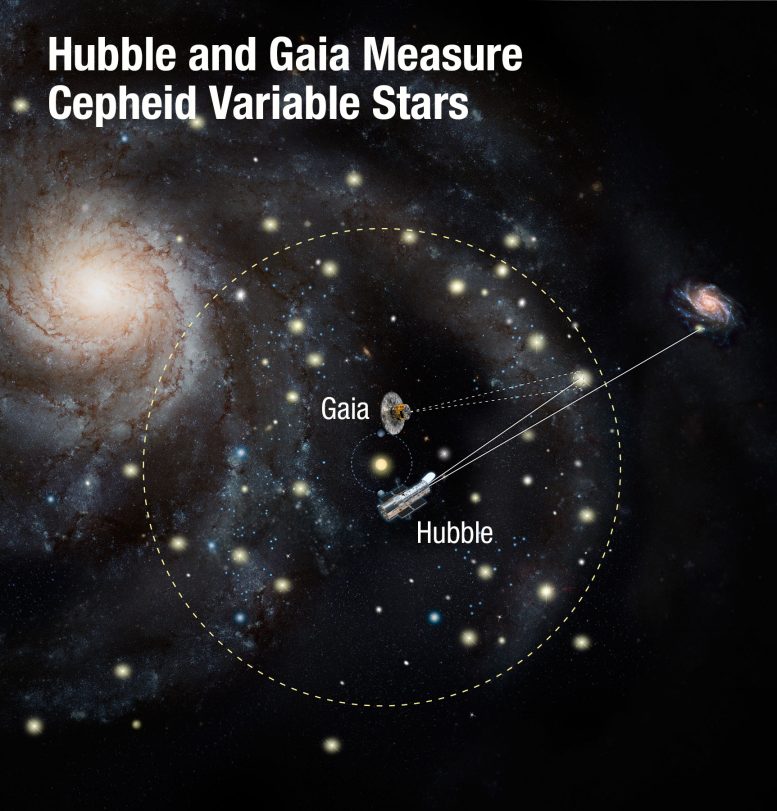
Using two of the world’s most powerful space telescopes — NASA’s Hubble and ESA’s Gaia — astronomers have made the most precise measurements to date of the universe’s expansion rate. This is calculated by gauging the distances between nearby galaxies using special types of stars called Cepheid variables as cosmic yardsticks. By comparing their intrinsic brightness as measured by Hubble, with their apparent brightness as seen from Earth, scientists can calculate their distances. Gaia further refines this yardstick by geometrically measuring the distances to Cepheid variables within our Milky Way galaxy. This allowed astronomers to more precisely calibrate the distances to Cepheids that are seen in outside galaxies. Credit: NASA, ESA, and A. Feild (STScI)
Using the power and synergy of two space telescopes, astronomers have made the most precise measurement to date of the universe’s expansion rate.
The results further fuel the mismatch between measurements for the expansion rate of the nearby universe, and those of the distant, primeval universe — before stars and galaxies even existed.
This so-called “tension” implies that there could be new physics underlying the foundations of the universe. Possibilities include the interaction strength of dark matter, dark energy being even more exotic than previously thought, or an unknown new particle in the tapestry of space.
Combining observations from NASA’s Hubble Space Telescope and the European Space Agency’s (ESA) Gaia space observatory, astronomers further refined the previous value for the Hubble constant, the rate at which the universe is expanding from the big bang 13.8 billion years ago.
But as the measurements have become more precise, the team’s determination of the Hubble constant has become more and more at odds with the measurements from another space observatory, ESA’s Planck mission, which is coming up with a different predicted value for the Hubble constant.
Planck mapped the primeval universe as it appeared only 360,000 years after the big bang. The entire sky is imprinted with the signature of the big bang encoded in microwaves. Planck measured the sizes of the ripples in this Cosmic Microwave Background (CMB) that were produced by slight irregularities in the big bang fireball. The fine details of these ripples encode how much dark matter and normal matter there is, the trajectory of the universe at that time, and other cosmological parameters.
These measurements, still being assessed, allow scientists to predict how the early universe would likely have evolved into the expansion rate we can measure today. However, those predictions don’t seem to match the new measurements of our nearby contemporary universe.
“With the addition of this new Gaia and Hubble Space Telescope data, we now have a serious tension with the Cosmic Microwave Background data,” said Planck team member and lead analyst George Efstathiou of the Kavli Institute for Cosmology in Cambridge, England, who was not involved with the new work.
“The tension seems to have grown into a full-blown incompatibility between our views of the early and late time universe,” said team leader and Nobel Laureate Adam Riess of the Space Telescope Science Institute and the Johns Hopkins University in Baltimore, Maryland. “At this point, clearly it’s not simply some gross error in any one measurement. It’s as though you predicted how tall a child would become from a growth chart and then found the adult he or she became greatly exceeded the prediction. We are very perplexed.”
In 2005, Riess and members of the SHOES (Supernova H0 for the Equation of State) team set out to measure the universe’s expansion rate with unprecedented accuracy. In the following years, by refining their techniques, this team shaved down the rate measurement’s uncertainty to unprecedented levels. Now, with the power of Hubble and Gaia combined, they have reduced that uncertainty to just 2.2 percent.
Because the Hubble constant is needed to estimate the age of the universe, the long-sought answer is one of the most important numbers in cosmology. It is named after astronomer Edwin Hubble, who nearly a century ago discovered that the universe was uniformly expanding in all directions—a finding that gave birth to modern cosmology.
Galaxies appear to recede from Earth proportional to their distances, meaning that the farther away they are, the faster they appear to be moving away. This is a consequence of expanding space, and not a value of true space velocity. By measuring the value of the Hubble constant over time, astronomers can construct a picture of our cosmic evolution, infer the make-up of the universe, and uncover clues concerning its ultimate fate.
The two major methods of measuring this number give incompatible results. One method is direct, building a cosmic “distance ladder” from measurements of stars in our local universe. The other method uses the CMB to measure the trajectory of the universe shortly after the big bang and then uses physics to describe the universe and extrapolate to the present expansion rate. Together, the measurements should provide an end-to-end test of our basic understanding of the so-called “Standard Model” of the universe. However, the pieces don’t fit.
Using Hubble and newly released data from Gaia, Riess’ team measured the present rate of expansion to be 73.5 kilometers (45.6 miles) per second per megaparsec. This means that for every 3.3 million light-years farther away a galaxy is from us, it appears to be moving 73.5 kilometers per second faster. However, the Planck results predict the universe should be expanding today at only 67.0 kilometers (41.6 miles) per second per megaparsec. As the teams’ measurements have become more and more precise, the chasm between them has continued to widen, and is now about four times the size of their combined uncertainty.
Over the years, Riess’ team has refined the Hubble constant value by streamlining and strengthening the “cosmic distance ladder,” used to measure precise distances to nearby and far-off galaxies. They compared those distances with the expansion of space, measured by the stretching of light from nearby galaxies. Using the apparent outward velocity at each distance, they then calculated the Hubble constant.
To gauge the distances between nearby galaxies, his team used a special type of star as cosmic yardsticks or milepost markers. These pulsating stars, called Cephied variables, brighten and dim at rates that correspond to their intrinsic brightness. By comparing their intrinsic brightness with their apparent brightness as seen from Earth, scientists can calculate their distances.
Gaia further refined this yardstick by geometrically measuring the distance to 50 Cepheid variables in the Milky Way. These measurements were combined with precise measurements of their brightnesses from Hubble. This allowed the astronomers to more accurately calibrate the Cepheids and then use those seen outside the Milky Way as milepost markers.
“When you use Cepheids, you need both distance and brightness,” explained Riess. Hubble provided the information on brightness, and Gaia provided the parallax information needed to accurately determine the distances. Parallax is the apparent change in an object’s position due to a shift in the observer’s point of view. Ancient Greeks first used this technique to measure the distance from Earth to the Moon.
“Hubble is really amazing as a general-purpose observatory, but Gaia is the new gold standard for calibrating distance. It is purpose-built for measuring parallax—this is what it was designed to do,” Stefano Casertano of the Space Telescope Science Institute and a member of the SHOES team added. “Gaia brings a new ability to recalibrate all past distance measures, and it seems to confirm our previous work. We get the same answer for the Hubble constant if we replace all previous calibrations of the distance ladder with just the Gaia parallaxes. It’s a crosscheck between two very powerful and precise observatories.”
The goal of Riess’ team is to work with Gaia to cross the threshold of refining the Hubble constant to a value of only one percent by the early 2020s. Meanwhile, astrophysicists will likely continue to grapple with revisiting their ideas about the physics of the early universe.
Reference: “Milky Way Cepheid Standards for Measuring Cosmic Distances and Application to Gaia DR2: Implications for the Hubble Constant” by Adam G. Riess, Stefano Casertano, Wenlong Yuan, Lucas Macri, Beatrice Bucciarelli, Mario G. Lattanzi, John W. MacKenty, J. Bradley Bowers, WeiKang Zheng, Alexei V. Filippenko, Caroline Huang and Richard I. Anderson, 12 July 2018, The Astrophysical Journal.
DOI:10.3847/1538-4357/aac82e
I am curious. Huge efforts are being made to measure the velocities in the universe, but relative to what reference point? the values cannot be understood properly without a stable reference. Have any efforts been made to identify the point of origin of the universe? We are a rapidly moving point in the universe whose motion is not understood fully. So how does anyone determine actual expansion rates without a fixed point of reference? The simple truth is without a fixed reference, we really do not know what happening.
In 1997, William. Masters at THE AMERICAN UNIVERSITY, in Washington D.C., wrote the paper STRETCHED AND NEW ZERO SPACETIME, A NEW MODLE OF GRAVITATION AND BLACK HOLE DYNAMICS. In it, he predicted and explained why the universe was expanding at an accelerating rate and described why Hubble’s Constant was wrong.
He described the black hole as a Super-compressed Bose-Einstein Condensate!
The quanta trapped inside were so compacted that they could not resonate at their natural frequencies. They were trying to express their natures but the constraint of gravitation prevented it.
Thus, in the Black Hole, quanta were converted into “quantum potentials”, while existing as only “quantum springs”, pushing out against gravitation but without effect.
Until that is, the Point of Diminishing Returns is reached!
Gravity, being a natural force, he says, must obey the Law of Diminishing Returns. With each unit of mass added to the Black Hole, the gravitational constraint increases one unit, but when Diminishing Returns is reached. Now each unit of mass added to the Black Hole will increase the gravitational constant, but at a slightly lesser amount than the previous unit of mass added.
With additional added mass, the expansive force of the quanta is increasing while Diminishing Returns is, relative to the expansive force, diminishing the gravitational constraint.
This weakening of the Black Hole’s gravitational constraint on the mass inside of the Black Hole causes the mass to expand slightly. The Black Hole begins to grow in size. This growth diminishes the Gravitational focus of the Black Hole causing the Event Horizon to move closer to the Black Hole.
Eventually, the added mass takes the Black Hole to the Point of Negative Returns, beginning the Big Bang. A process he says occurs in Near-Zero Spacetime, and so takes hundreds of thousands of years to occur.
Masters claims the Universe is expanding at an accelerating rate because the laws of Force and Motion govern the universe’s expansion.
Masters views the Black Hole as onion-like, with varying layers possessing varying amounts of potential kinetic energy.
All movement is the result of a Net force, with an equal and opposite force.
The outer layers of Quanta are actually pushing off the inner layers of quanta. Like a basketball Player pushing off the court’s flooring to jump up for a shot.
The first outer layer of quanta is pushing off the second layer, and so takes some of the second layers kinetic energy. The second layer boosts the layer above it. Likewise, the second layer is pushing off the third layer of quanta, and taking some of its kinetic energy. The third layer of quanta are pushing back against the fourth layer and so on.
As you go into the Black Hole, each layer of quanta leaves the Black Hole during the Big Bang with slightly less kinetic energy than the next outermost layer of quanta above it.
Eventually, as we travel inward through the layers of quant we will come to the Midian Quantum Layer. Here the inward pushing of the outgoing quanta is equal to the outward pushing of the inner layers of quanta, and so this layer will never leave the black hole. This is the black Hole Remnant that will be left after the Big Bang has ended.
As the expansion of the Universe is magnitudes greater than the speed of light, this creates a simple explanation for its acceleration.
The last layer of quanta to leave the black hole has so little kinetic energy that it quickly falls back into the black hole. The next layer of quanta have a little more kinetic energy and so go a little further away, but they also run out of energy and fall back into the black hole.
Like the ball you toss into the air, gravitation wins out.
This process of quanta falling back into the black hole continues until we come to the ESCAPE VELOCITY LAYER. These quanta have enough kinetic energy to escape the gravitation of the Black Hole, however, when they cross the gravitational boundary, they will exhaust the last of their kinetic energy and come to a full stop and remain there forever.
To an outside observer, these quanta were seen as slowing down as they moved away from the Black Hole.
The next outer most layer of quanta has slightly more kinetic energy than the Escape Velocity Layer, and so after crossing the gravitational boundary stops slowing down and now moves at a steady speed equal to the kinetic energy that remains.
As we move through the outer layers beyond these, their kinetic energy levels grow and so the speed they move at after crossing the gravitational boundary is greater than the inner layers of quanta.
As we continue to move outward through the layers of quanta, we will reach the 2X ESCAPE VELOCITY layer.
These quanta have twice the kinetic energy needed to escape the gravitation. As such, they lose their kinetic energy at a rate that is half the rate that gravity is diminishing at. Thus, to an outside observer, these quanta have been moving at a fixed rate of speed. Which they maintain after crossing the gravitational boundary.
Outside of the 2X layer, are the SUPER X layers. These quanta have more than twice the energy needed to escape the Black Hole, as such, for each equal length they travel, their kinetic energy is growing relative to the pull of gravitation from the Black Hole. As such, their speed is increasing as they travel away from the Black Hole.
When they eventually cross the gravitational boundary, there will be no more retarding gravitation from the black hole and as such, they now will fully express their kinetic energy as a steady set speed equal to that kinetic energy left after their long journey.
There is now a very simple way to calculate Hubble’s Constant, by inputting to an equation, the numerical value of Pi and the speed of light (C) from Maxwell’s equations, and the value of a parsec. NO space probe measurements (with their inevitable small measuring / interpretation errors) are now required. Hubble’s Constant is ‘fixed’ at 70.98047 PRECISELY. This maths method removes the errors / tolerances that is always a part of attempting to measuring something as ‘elusive’ as Hubble’s Constant. This has very deep implications for theoretical cosmology.
The equation to perform this is :- 2 X a meg parsec X light speed (C). This total is then divided by Pi to the power of 21. This gives 70.98047 kilometres per sec per meg parsec.
The equation to perform this can also be found in ‘The Principle of Astrogeometry’ on Amazon Kindle Books. This also explains how the Hubble 70.98047 ‘fixing’ equation was found. David.