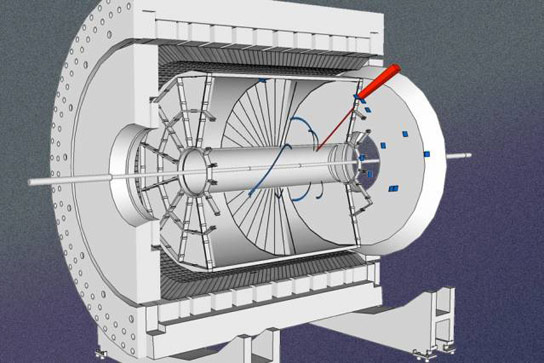
The STAR detector, used in the researchers’ experiment, measures the energy and angle of the electron from the W boson decay produced in the proton collision. Credit: STAR Collaboration
Using the STAR detector at Brookhaven National Laboratory, scientists have identified a new method sensitive to the antiquark spin, which can shed light on where these quarks and antiquarks come from.
This fundamental question has been a longstanding mystery in particle physics, although it was once thought that the answer would be fairly straightforward: The spin of a proton’s three subatomic particles, called quarks, would simply add up to produce its total spin.
But a series of experiments in the 1980s threw this theory for a loop, proving that the spins of the quarks are only partially responsible for the proton’s overall spin. Thus emerged what physicists now refer to as the “proton spin crisis,” prompting a decades-long search for the missing pieces, or contributors, to a proton’s spin.
Now an international team of more than 300 researchers, including MIT physicists, has placed new constraints on the spin of the proton’s antiquarks — the antiparticles of quarks that are thought to arise when the bonds between quarks break. The researchers say these measurements may help to identify the antiquark’s role in the proton’s spin, as well as the mechanism by which antiquarks are produced.
“We’d like to understand the spin contributions of the subatomic particles inside the proton, to learn something fundamental about their interactions,” says Justin Stevens, a postdoc in MIT’s Laboratory for Nuclear Science (LNS). “Now we have a new method sensitive to the antiquark spin, which can shed light on where these quarks and antiquarks come from.”
Stevens and Jan Balewski, a research scientist in the LNS, led the analysis of more than 1 billion recorded proton collisions produced by the Relativistic Heavy Ion Collider (RHIC), a particle accelerator at Brookhaven National Laboratory. Using the facility’s STAR detector, which tracks the particles produced by each collision, the team identified 3,500 proton collisions that produced a W boson — an elementary particle that, when generated, temporarily inherits the spin of a proton’s antiquark.
“We measured the decay product of the W boson, and from this, we could infer the spin of the antiquark, and how it relates to the spin of the mother proton,” Balewski explains. “It turns out the antiquark polarization is marginal, and contributes very little to the polarization of the proton.”
Stevens, Balewski, and their collaborators publish their experimental results today in the journal Physical Review Letters.
When protons collide
According to the Standard Model of particle physics, the proton is a composite particle composed of three quarks, each of a distinct type, or “flavor”: two “up” quarks, and one “down” quark. These quarks are bound together by particles called gluons which, when temporarily broken, are thought to give rise to pairs of short-lived quarks and antiquarks. Since a proton’s spin cannot be fully explained by the spin of its quarks, physicists have looked to other possible contributors, such as the spins of gluons and antiquarks, or their orbital motion inside the proton.
Stevens, Balewski, and their colleagues identified the antiquarks by measuring the decay of W bosons following collisions of polarized protons. As Balewski explains it, at any given moment, a polarized or spinning proton contains pairs of quarks and antiquarks that “pop out and disappear, pop out and disappear.”
“When they show up, the spin of the proton is passed to those antiquarks to some degree,” Balewski says. “Now this antiquark, for a fraction of a second, shows up in the proton exactly at the moment when this proton collides with this other proton, and the W boson is produced.”
The researchers observed a significant difference in the number of W bosons produced when the proton’s spin was oriented in the same direction as its motion compared to cases where the proton’s spin was oriented in the opposite direction. The antiquark spin was then inferred by measuring this difference for various orientations of the electrons produced as the W boson decays.
Exploring antiquarks’ origins
The researchers’ results provide significant new constraints on the spin of the proton’s antiquarks, which contribute a small fraction to the total spin of the proton. In addition, they observed a slightly larger-than-expected spin effect for a subset of up-flavored antiquarks compared to down-flavored antiquarks. Stevens says this asymmetry in antiquark spin may help to identify how a proton’s antiquarks arise in the first place.
“The naive picture of how these quark/antiquark pairs pop in and out of existence is that gluons split to form these pairs,” Stevens says. “But if that were the case, you’d expect to get equal numbers of up- and down-flavored quarks. Our measurements provide some new information, which could tell us something about how these quarks and antiquarks are produced.”
Marco Stratmann, a staff scientist at the Institute for Theoretical Physics at the University of Tubingen, says the group’s data “provide us with ‘snapshots’ of the proton’s spin and flavor structure. … In [future] analyses of the proton’s spin structure, this data will provide a novel and particularly clean probe of the up and down antiquark polarizations, largely free of theoretical uncertainties.”
“We’re tightening up the constraints on what this antiquark polarization looks like,” Stevens says. “And with future data, that constraint will get even better.”
Reference: “Measurement of Longitudinal Spin Asymmetries for Weak Boson Production in Polarized Proton-Proton Collisions at RHIC” by L. Adamczyk et al. (STAR Collaboration), 13 August 2014, Physical Review Letters.
DOI: 10.1103/PhysRevLett.113.072301
This research was supported in part by the National Science Foundation and the U.S. Department of Energy.
Why do protons need to be made from smaller particles to have spin? Where would gluons come from and why would nature make this interaction beteween quarks and gluons? it sounds like terrible reverse engineering. they invented this model of how they think protons work, because they cannot explain the strong force. hese things aren’t affected by things like angular momentum and forces that affect the objects that atoms create. these particles are deefined by the surface tension that they create with aether.
here’s what they get up to at MIT http://video.mit.edu/watch/the-origin-of-mass-and-the-feebleness-of-gravity-9082/
He looks like he knows that he’s full of t-i-h-s
Hello Sir! Once the concept of Mass-energy relationship is accepted and it is found to be inter-convertible , there should not be any doubt that Proton should be the ultimate smallest particle. After all every particle should come from some energy conversion. Hence the discovery of quarks makes that constituents of Proton are Up, Up and Down quarks and that of Neutrons are Up, down and Down quarks. Yes, quarks are particles in real sense since they are localized and occupy a definite space unlike Bosons (Radiation Particles which are superimposing on one another called Photons). They are like ghosts and now the ghosts have to be materialized as solids as quarks. But,three quarks won’t account for the entire mass of one Proton or Neutron. The huge deficiency in the sum of the masses should be accounted somehow. This is called another type of force called strong forces of nucleus which will overwhelm the trivial electron force of repulsion of positive protons packed in the nucleus. The force particles are called gluons and the stability comes with only three of such forces and they are red gluon, blue gluon and green gluon. (Red, Blue and Green are named only for labelling purpose as we do in dual forces like positive and negative in electrons and positrons) Still fragments of quarks may be possible but the Higgs Boson which holds these ghosts to configure cannot capture them with less fragments. But Higgs Boson exists as ghosts as long as it wanders and once it encounters any equal partners of energy like gluons and electric field to materialize. These ghost particles are highly massive in some kinds as in the case of weak field of W+,W- and Z Bosons. The weak forces cling on to the periphery of heavy nuclei and once comes out it transforms into solid electrons and quarks. You have seen radioactivity where alpha particles which are nothing but Helium Nuclei, generated from this ghost bosons and electrons (Beta particles) and gamma photons. Thus energy is exchanged for particles in the exchange. Even the strong forces will exchange for real particles once they are stretched too much from their position. Thus divide the energy also into small constituents, something very strong and something very feeble like gravity, where the size doesn’t matter. Only in material world size matters. Even Dark Matter and Dark Energy may be energy ensembles of ghosts, but prevalent in the universe to the extent of 96%. Thank You.
There is no such thing as energy (it is only a measurement), so there can’t be energy-mass conversion. This experiment proves that light is a reaction that aether has, caused by atomic activity. Mass is not limited by the speed of light. Light and all other electro magnetic radiation is a reaction that is pulled forward at the same speed–it just has different energy levels. Light is not a particle and has nothing to do with mass.
Claiming there is a Higgs feild is similar to claiming that the reason amebas cannot evolve into multi celled creatures is because there is an ameba feild that keeeps therm this way. There is EVERYTHING in the universe, but conveiniently there is an ameba field………….