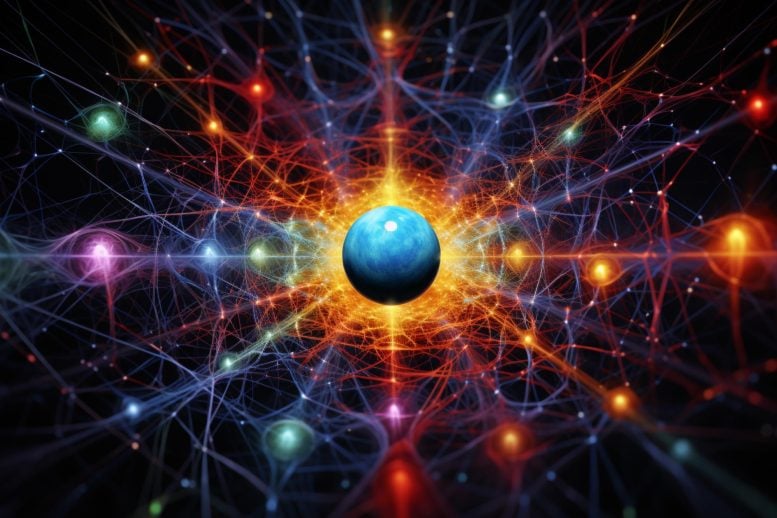
Quantum physicists from Hiroshima University have revealed that the results of quantum measurements are fundamentally tied to the interaction dynamics between the measuring device and the system, challenging traditional views of fixed physical properties and suggesting that reality is shaped by the context of these interactions. Their findings point to a need to rethink the interpretation of quantum experimental data.
Whenever measurement precision nears the uncertainty limit set by quantum mechanics, the results become dependent on the interaction dynamics between the measuring device and the system. This finding may explain why quantum experiments often produce conflicting results and may contradict basic assumptions regarding physical reality.
Research Analysis and Findings
Two quantum physicists from Hiroshima University recently analyzed the dynamics of a measurement interaction, where the value of a physical property is identified with a quantitative change in the meter state. This is a difficult problem, because quantum theory does not identify the value of a physical property unless the system is in a so-called “eigenstate” of that physical property, a very small set of special quantum states for which the physical property has a fixed value.
The researchers solved this fundamental problem by combining information about the past of the system with information about its future in a description of the dynamics of the system during the measurement interaction, demonstrating that the observable values of a physical system depend on the dynamics of the measurement interaction by which they are observed.
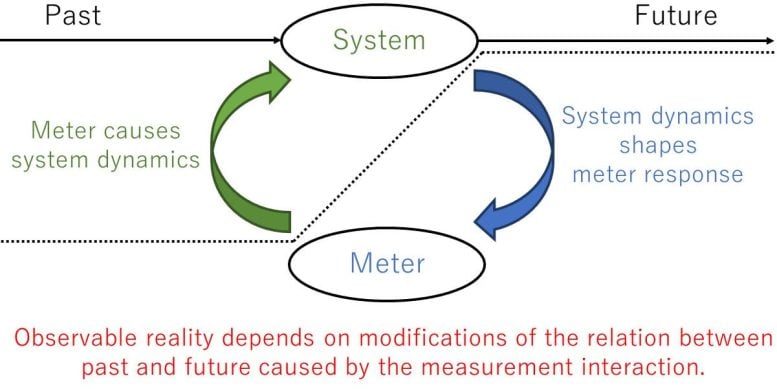
According to quantum theory, measurement results are shaped by the changes in the relation between the past and the future of a system caused by the measurement interaction. Credit: Tomonori Matsushita and Holger F. Hofmann, Hiroshima University
The team recently published the results of their study in the journal Physical Review Research.
“There is much disagreement about the interpretation of quantum mechanics because different experimental results cannot be reconciled with the same physical reality,” said Holger Hofmann, professor in the Graduate School of Advanced Science and Engineering at Hiroshima University in Hiroshima, Japan.
“In this paper, we investigate how quantum superpositions in the dynamics of the measurement interaction shape the observable reality of a system seen in the response of a meter. This is a major step towards explaining the meaning of “superposition” in quantum mechanics,” said Hofmann.
Superposition and Physical Reality
In quantum mechanics, a superposition describes a situation in which two possible realities seem to co-exist, even though they can be distinguished clearly when an appropriate measurement is performed. The analysis of the team’s study suggests that superpositions describe different kinds of reality when different measurements are performed. The reality of an object depends on the object’s interactions with its surroundings.
“Our results show that the physical reality of an object cannot be separated from the context of all its interactions with the environment, past, present, and future, providing strong evidence against the widespread belief that our world can be reduced to a mere configuration of material building blocks,” said Hofmann.
According to quantum theory, the meter shift that represents the value of the physical property observed in a measurement depends on the dynamics of the system caused by the fluctuations of the back-action by which the meter disturbs the state of the system. Quantum superpositions between the different possible system dynamics shape the meter response and assign specific values to it.
The authors further explained that the fluctuations in the system dynamics depend on the strength of the measurement interaction. In the limit of weak interactions, the fluctuations of the system dynamics are negligibly small and the meter shift can be determined from the Hamilton-Jacobi equation, a classical differential equation expressing the relation between a physical property and the dynamics associated with it.
When the measurement interaction is stronger, complicated quantum interference effects between different system dynamics are observed. Fully resolved measurements require a complete randomization of the system dynamics. This corresponds to a superposition of all possible system dynamics, where quantum interference effects select only those components of the quantum process that correspond to the eigenvalues of the physical property.
Eigenvalues are the values that textbook quantum mechanics assigns to measurement outcomes – precise photon numbers, spin up or spin down, and so forth. As the new results show, these values are a result of the complete randomization of the dynamics. Different values need to be considered when the system dynamics is not completely randomized by the measurement.
Implications for Understanding Quantum Measurements
Interestingly, this observation provides a new perspective on the use of measurement outcomes in descriptions of reality. It is common to assume that localized particles or integer spin values are measurement-independent elements of reality, but these research results suggest that these values are only created by quantum interferences in sufficiently strong measurements. Our understanding of the meaning of experimental data may be in need of a fundamental revision.
Hofmann and his team look forward to further clarifying the contradictory results observed in many quantum experiments. “Context-dependent realities can explain a wide range of seemingly paradoxical quantum effects. We are now working on better explanations of these phenomena. Ultimately, the goal is to develop a more intuitive understanding of the fundamental concepts of quantum mechanics that avoids the misunderstandings caused by a naive belief in the reality of microscopic objects,” said Hofmann.
Reference: “Dependence of measurement outcomes on the dynamics of quantum coherent interactions between the system and the meter” by Tomonori Matsushita and Holger F. Hofmann, 31 July 2023, Physical Review Research.
DOI: 10.1103/PhysRevResearch.5.033064
The study was funded by the Japan Science and Technology Agency.
If the result of these studies carried out by these two scientists is correct, anything could be anything.
That is the core of the problem. It is an untestable model proposal, so the simpler model of “anything can be anything” is equally relevant.
Yeah because there is a singularity of states in the device and the system in the form of information on the meter. Its entanglement.
On the contrary, they explicitly study back action of pure, unentangled states.
This goes along the lines I have always thought. An a particle’s position can’t be determined precisely because it is moving so fast. Our instruments take snap shots of the particle, and each snap shot shows the particle in a different place. However if you could take 100 Trillion snap shots in a second, then you might have a chance to see the true motion of the particle. Right now we have nothing that can sample at this high of a frame rate. In the future, we might develop this ability, and only them will we see the true motion of these particles. If a particle is moving around an atom 30 Trillion times a second, you would need to sample at 3-4 times that rate to see a clear indication of the true motion. Yes, the instruments used to observe do very much affect the readings. “The act of observation changes the results seen.”.
This is completely incorrect due to the Heisenberg Uncertainty Principle, which puts a limit on how precise our simultaneous knowledge of a particle’s position and momentum (or other similar combinations of properties) can be. The limit is not actually on our knowledge, but is a result of the fact that there is an inherent uncertainty in the actual value of the combination of a particle’s position and momentum, as expressed by the following equation:
Δx Δp ≥ ħ/2
This is one of the most famous equations in quantum mechanics.
The Heisenberg Uncertainty Principle is very well illustrated by the following joke:
A particle physicist is speeding down the highway when she gets pulled over by a police officer. The cop says: “Ma’am, do you have any idea of how fast you were going?”
The physicist replies: “No, but I know exactly where I am.”
This is quantum physics which means that fields are the fundamental entities and particles are resonant ripple excitations with behavior such as a tendency to localize et cetera. Due to the uncertainty principle, and demonstrated by the space filling particle trajectories of the Feynman path integral method, there is no particle straight line “true motion” of the ripples.
If you read the paper that you want to comment on, it notes that: “However, quantum fluctuations play a significant role in free space particle propagation once we attempt to control the trajectories with a precision higher than the one given in Eq. (18). It might be worth noting that this is the regime in which superpositions of position and momentum can be used to demonstrate that quantum particles do not propagate in straight lines, as shown in a recent experiment [36–38].”
But what does this mean for the health of Schrödinger’s cat? Is it dead, alive, or just cat-napping? Should we call a vet?
Sounds like De Broglie-Bohm Pilot Waves to me
Its a wonderful short explanation :this been y reading habits 🙂 quantum mechanics. Energy .movement.ingredients.
Quantum doesn’t always be the star sun power or wave. Its a 🖥 👨💻 👩💻 🖲 💻 computer that can see beyond a micro scope…
Liked the article. Bring back some where is the 😂🤣😆men in black. Now that was a funday…
If I am understanding this correctly, the value detected is the result of an interaction between the observer and the observed. We are not observing a fixed state, but rather a dynamic probability field, that is influenced by the act of observation. Since that interaction can be different each time, so are the results.
its a system it does not depend on your observation it depend on its workability
They suggest something like that, but does not provide any observations that suggest our measurements – which works – should be replaced by their model.
That’s just it must be observed seeing it until it’s noticed that’s the moment
they using quantum to solve that problem
I don’t understand.
If you are interested, reading the paper is a good start.
“This is a difficult problem, because quantum theory does not identify the value of a physical property unless the system is in a so-called “eigenstate” of that physical property, a very small set of special quantum states for which the physical property has a fixed value.”
That is absolutely not true. The Schrodinger equation describes time dependent quantities as well. They’re just a lot harder to observe due to the rate at which they change. This, in fact, is what won the Nobel Prize this year.
Either you are confused or these authors are. I suspect you. A measurement involves the thing you measure and the thing you use to measure it. The outcome depends on both. The reason you can get seemingly contradictory results between two measurements is that those measuring devices measure complementary information. That means properties that cannot be measured simultaneously, like position (a particle like property) and momentum (a wave like property). No experiment ever gives a contradictory result because you can’t do both of those measurements in one go. This has been known since the 1920’s.
That is a good point!
Their model, adding back action, isn’t even strictly classical quantum mechanics at heart, likely for smilar obfuscation reasons. They use the Hamilton–Jacobi equation to get as close as possible to classical “want non-complementary properties” physics. “The Hamilton–Jacobi equation is the only formulation of mechanics in which the motion of a particle can be represented as a wave.” They may not be studying particle state behavior at all, though I couldn’t really tell without them suggesting experiments.
This is a super Wigner Friends paradox, where the reality of the Friend is not the Same as Wigner, both realities coexist, but is not garanteed from Wigners point of view that the reality of his friend is indeed the same. In the factual the friend will die agreeing that the reality you both see in the measurement is equal, but this is also your `relative` quantum bubble, where everything will always make sense from your senses (human measurement).
The paper does not study such models. Maybe you should read it instead of speculate what it says.
The paper analyses a strawman where the standard interpretation of measurement results are changed to one suggesting back-action effects which the analysis then of course ‘find’. No actual measurements and back-action effects are presented, but meanwhile the standard interpretation works. The paper implicitly admits that since they accept “weak measurements” that observe classical non-quantum physics values.
In a more general context, beyond the futile work with non-testable “interpretations”, we know that measurements of quantum field effects – observing reality – such as entanglement can be non-local, even without considering supposed back-actions. We just have to be careful what we measure.
I’ll add that “futile” is the general result, so far, of proposing non-theory (non-testable) models. If we can observe back-action effects this work may have future relevance.