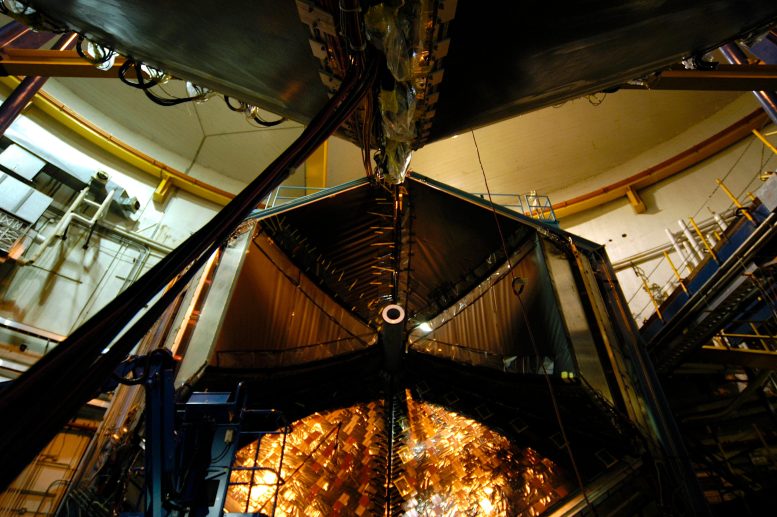
Jefferson Lab’s CEBAF Large Acceptance Spectrometer in Experimental Hall B. Credit: DOE’s Jefferson Lab
New findings from Jefferson Laboratory shed light on the process of forming strange matter from ordinary matter.
Nuclear physicists have made a groundbreaking discovery through their unique analysis of experimental data. For the first time ever, they have observed the production of lambda particles, also known as “strange matter,” through a process called semi-inclusive deep inelastic scattering (SIDIS). The data obtained also suggests that the building blocks of protons, quarks, and gluons can sometimes march through the nucleus of an atom in pairs referred to as diquarks. The experiment was carried out at the Thomas Jefferson National Accelerator Facility, which is run by the U.S. Department of Energy.
This achievement has been the culmination of many years of hard work. The data that was used in this study was originally gathered in 2004. Lamiaa El Fassi, who is currently serving as an associate professor of physics at Mississippi State University and is the lead researcher of this project, initially analyzed these data while she was working on her thesis project to obtain her graduate degree on a different topic.
Nearly a decade after completing her initial research with these data, El Fassi revisited the dataset and led her group through a careful analysis to yield these unprecedented measurements. The dataset comes from experiments in Jefferson Lab’s Continuous Electron Beam Accelerator Facility (CEBAF), a DOE user facility. In the experiment, nuclear physicists tracked what happened when electrons from CEBAF scatter off the target nucleus and probe the confined quarks inside protons and neutrons. The results were recently published in Physical Review Letters.
“These studies help build a story, analogous to a motion picture, of how the struck quark turns into hadrons. In a new paper, we report first-ever observations of such a study for the lambda baryon in the forward and backward fragmentation regions,” El Fassi said.
In like a lambda, out like a pion
Like the more familiar protons and neutrons, each lambda is made up of three quarks.
Unlike protons and neutrons, which only contain a mixture of up and down quarks, lambdas contain one up quark, one down quark, and one strange quark. Physicists have dubbed matter that contains strange quarks “strange matter.”
In this work, El Fassi and her colleagues studied how these particles of strange matter form from collisions of ordinary matter. To do so, they shot CEBAF’s electron beam at different targets, including carbon, iron, and lead. When a high-energy electron from CEBAF reaches one of these targets, it breaks apart a proton or neutron inside one of the target’s nuclei.
“Because the proton or neutron is totally broken apart, there is little doubt that the electron interacts with the quark inside,” El Fassi said.
After the electron interacts with a quark or quarks via an exchanged virtual photon, the “struck” quark(s) begins moving as a free particle in the medium, typically joining up with other quark(s) it encounters to form a new composite particle as they propagate through the nucleus. And some of the time, this composite particle will be a lambda.
But the lambda is short-lived – after formation, it will swiftly decay into two other particles: a pion and either a proton or neutron. To measure the different properties of these briefly created lambda particles, physicists must detect its two daughter particles, as well as the beam electron that scattered off the target nucleus.
The experiment that collected this data, EG2, used the CEBAF Large Acceptance Spectrometer (CLAS) detector in Jefferson Lab’s Experimental Hall B. These recently published results, “First Measurement of Λ Electroproduction off Nuclei in the Current and Target Fragmentation Regions,” are part of the CLAS collaboration, which involves almost 200 physicists worldwide.
SIDIS
This work is the first to measure the lambda using this process, which is known as semi-inclusive deep inelastic scattering, in the forward and backward fragmentation regions. It’s more difficult to use this method to study lambda particles, because the particle decays so quickly, it can’t be measured directly.
“This class of measurement has only been performed on protons before, and on lighter, more stable particles,” said coauthor William Brooks, professor of physics at Federico Santa María Technical University and co-spokesperson of the EG2 experiment.
The analysis was so challenging, it took several years for El Fassi and her group to re-analyze the data and extract these results. It was her thesis advisor, Kawtar Hafidi, who encouraged her to pursue the investigation of the lambda from these datasets.
“I would like to commend Lamiaa’s hard work and perseverance in dedicating years of her career working on this,” said Hafidi, associate laboratory director for physical sciences and engineering at Argonne National Lab and co-spokesperson of the EG2 experiment. “Without her, this work would not have seen fruition.”
“It hasn’t been easy,” El Fassi said. “It’s a long and time-consuming process, but it was worth the effort. When you spend so many years working on something, it feels good to see it published.”
El Fassi began this lambda analysis when she herself was a postdoc, a couple of years prior to becoming an assistant professor at Mississippi State University. Along the way, several of her own postdocs at Mississippi State have helped extract these results, including coauthor Taya Chetry.
“I’m very happy and motivated to see this work being published,” said Chetry, who is now a postdoctoral researcher at Florida International University.
Two for one
A notable finding from this intensive analysis changes the way physicists understand how lambdas form in the wake of particle collisions.
In similar studies that have used semi-inclusive deep inelastic scattering to study other particles, the particles of interest usually form after a single quark was “struck” by the virtual photon exchanged between the electron beam and the target nucleus. But the signal left by lambda in the CLAS detector suggests a more packaged deal.
The authors’ analysis showed that when forming a lambda, the virtual photon has been absorbed part of the time by a pair of quarks, known as a diquark, instead of just one. After being “struck,” this diquark went on to find a strange quark and forms a lambda.
“This quark pairing suggests a different mechanism of production and interaction than the case of the single quark interaction,” Hafidi said.
A better understanding of how different particles form helps physicists in their effort to decipher the strong interaction, the fundamental force that holds these quark-containing particles together. The dynamics of this interaction are very complicated, and so is the theory used to describe it: quantum chromodynamics (QCD).
Comparing measurements to models of QCD’s predictions allows physicists to test this theory. Because the diquark finding differs from the model’s current predictions, it suggests something about the model is off.
“There is an unknown ingredient that we don’t understand. This is extremely surprising since the existing theory can describe essentially all other observations, but not this one,” Brooks said. “That means there is something new to learn, and at the moment, we have no clue what it could be.”
To find out, they’ll need even more measurements.
Data for EG2 were collected with 5.014 GeV (billion electron-volt) electron beams in the CEBAF’s 6 GeV era. Future experiments will use electron beams from the updated CEBAF, which now extend up to 11 GeV for Experimental Hall B, as well as an updated CLAS detector known as CLAS12, to continue studying the formation of a variety of particles, including lambdas, with higher-energy electrons.
The upcoming Electron-Ion Collider (EIC) at DOE’s Brookhaven National Laboratory will also provide a new opportunity to continue studying this strange matter and quark pairing structure of the nucleon with greater precision.
“These results lay the groundwork for upcoming studies at the upcoming CLAS12 and the planned EIC experiments, where one can investigate the diquark scattering in greater detail,” Chetry said.
El Fassi is also a co-spokesperson for CLAS12 measurements of quark propagation and hadron formation. When data from the new experiments is finally ready, physicists will compare it to QCD predictions to further refine this theory.
“Any new measurement that will give novel information toward understanding the dynamics of strong interactions is very important,” she said.
Reference: “First Measurement of Λ Electroproduction off Nuclei in the Current and Target Fragmentation Regions” by T. Chetry et al. (CLAS Collaboration), 4 April 2023, Physical Review Letters.
DOI: 10.1103/PhysRevLett.130.142301
They ought to do away with the funny names. Strange has a meaning and connotation in english. Atoms and subatomic particles know nothing of english and it’s connotations. the scientists studying this are doing nothing more than making click bait and the journalists who report on their work lap it up. Those of us educated enough to understand sub-atomic particles are not impressed. So that leaves the ignorant masses to make whatever assumptions the connotations engender.
These scientists were doing science, which is very useful work with high return on investment.
But maybe you are just trolling.
If they could stabilize these particles, keep them together, and accumulate them, there might be some very interesting material science here.
Clickbait headlines do more harm than good. I skip articles and videos on QM that say things like “weird” or “strange”, with the single exception of referring to Einstein’s “spooky action at a distance”. Then this subject, the actual words are misused to write clickbait and confuse the public. Then there is all this nonsensical fluff to pad for ads. Why even write this stuff when ChatGPT will rewrite any other article for you. You’ve already seen the “flattening” or the “shallowing” of the internet. This is more of the same. Most of this country is flattening or shallowing in intelligence.
There was nothing wrong with the title.
But maybe you are just trolling.
The material hierarchy and its interaction ways are vital for understanding the particle decays. It is believed with the improvement of theoretical level and the progress of science and technology, the understanding for the interaction between topological vortices and their twin antivortices will usher a vast frontiers in physics.
Most people don’t read physics-speak. The real question in most people’s minds is probably, “What was actually accomplished here?” Because it reads like a bunch of recipes for NOTHING BURGERS. In other words, SO WHAT? What new product/propellent/transporter beam/fusion reactor/do-hickey will this information help to create? The answer is nothing. Nada. Nil. Zero. Zip.
I’m sorry, but the Hadron collider in particular has been one of the biggest wastes of funding in the history of mankind. Nothing it’s produced has improved life on Earth one iota. Where are our anti-gravity spaceships from finding the Higgs Boson? That’s right. It’s just a swirl on a cloud chamber that matches the shape some prediction made (probably by sheer coincidence) as it’s done NOTHING for us whatsoever except to watch a bunch of pseudo-scientists congratulate themselves on solving the fundamental mystery of quantum theory while in reality it hasn’t changed a thing. We still don’t fundamentally understand gravity or how to make a true artificial form of it (or its opposite), not just centripetal force. How much money is spent on this crap? The only quantum event that ever changed anything was in Alamogordo, New Mexico.
Worse yet, combining Einstein and Quantum theory results in infinity (meaning something is fundamentally WRONG in one of the two or both; given how ridiculous quantum mechanic formulas are compared to the simplicity of Einstein’s equations, I’d have to say the massive error is in the Quantum realm. Perhaps we could send Ant-Man and the Wasp there to find out what it is? Because as I see it, nothing is going to change. Money that could be better used (like going to Mars to look for underground signs of aliens…even the bacterial type) is just being wasted.
These scientists were doing science, which is very useful work with high return on investment. And we have enough money to walk and chew gum – invest in more than one method – at the same time. That’s why our capabilities and well being grow exponentially.
But maybe you are just trolling.
As for the Large Hadron Collider – there are others colliding protons and atoms – it has been crucial for testing and extending the modern description of particles. It is easy to understand when you notice that it netted Nobel Prizes.
That relativistic quantum field theory is effective and renormalizable is not a problem. Since there isn’t any “fundamental theory of nature” apart from it, you have to use observations to extract predictions to test. And despite infinities it has been the most precise theory ever devised (apart from phylogenies of biology, which due to its combinatorics beats everything else). This is well understood by the scientists using these theories.
Renormalization [from Wikipedia]:
“Renormalization was first developed in quantum electrodynamics (QED) to make sense of infinite integrals in perturbation theory. Initially viewed as a suspect provisional procedure even by some of its originators, renormalization eventually was embraced as an important and self-consistent actual mechanism of scale physics in several fields of physics and mathematics.
Today, the point of view has shifted: on the basis of the breakthrough renormalization group insights of Nikolay Bogolyubov and Kenneth Wilson, the focus is on variation of physical quantities across contiguous scales, while distant scales are related to each other through “effective” descriptions. All scales are linked in a broadly systematic way, and the actual physics pertinent to each is extracted with the suitable specific computational techniques appropriate for each. Wilson clarified which variables of a system are crucial and which are redundant.”
Evolution [from TalkOrigins]:
“Nevertheless, a precision of just under 1% [in independent measurements of the gravitational constant G] is still pretty good; it is not enough, at this point, to cause us to cast much doubt upon the validity and usefulness of modern theories of gravity. However, if tests of the theory of common descent performed that poorly, different phylogenetic trees, as shown in Figure 1, would have to differ by 18 of the 30 branches! In their quest for scientific perfection, some biologists are rightly rankled at the obvious discrepancies between some phylogenetic trees (Gura 2000; Patterson et al. 1993; Maley and Marshall 1998). However, as illustrated in Figure 1, the standard phylogenetic tree is known to 38 decimal places, which is a much greater precision than that of even the most well-determined physical constants. For comparison, the charge of the electron is known to only seven decimal places, the Planck constant is known to only eight decimal places, the mass of the neutron, proton, and electron are all known to only nine decimal places, and the universal gravitational constant has been determined to only three decimal places.”
An update on LHC:
“”Around 2040-2045, we will have taken away all the substance of the precision possible with the LHC,” he said.
“It will be time to move on to something much more powerful, much brighter, to better see the contours of the physics that we are trying to study.””
“The FCC would form a new circular tunnel under France and Switzerland, 91 kilometres (56.5 miles) long and about five metres (16 feet) in diameter.
“The goal of the FCC is to push the energy and intensity frontiers of particle colliders, with the aim of reaching collision energies of 100 tera electron volts, in the search for new physics,” CERN says.”
“After carrying out a theoretical analysis, “we are now embarking for the first time on field activities” to study potential environmental issues, he said, with seismic and geotechnical studies to follow.
Once the feasibility studies are completed, CERN’s member states—22 European countries plus Israel—will decide in the next five to six years on whether to build the FCC.
The FCC would accelerate electrons and positrons until 2060, and then hadrons until 2090, as it seeks answers to many remaining questions of fundamental physics, with about 95 percent of the mass and energy of the universe still a mystery.”
A snapshot on physics uses in society:
“”The benefits of our research are extremely important,” said Malika Meddahi, CERN’s deputy director for accelerators and technology, citing as examples medical imaging and the fight against tumours.
Janot agreed: “The day the electron gun was invented, it was the beginning of accelerators; we didn’t know it was going to give rise to television. The day general relativity was discovered, we didn’t know it was going to be used to run GPS.””
You know where Physicists can find a large quantity of strange matter to study? In between the ears of radical politicians. Yes, a very small joke. As small as the period at the end of this sentence.
You know where Physicists can find a large quantity of Strange Matter to study. Between the ears of radical politicians. Yes, a minuscule joke. About the size of the period at the end of this sentence.
How come physicists haven’t expanded the use of elements. Which is possible.
How do you mean? What is “use” and what is “expansion” here?
There is a long ongoing extension of the element table. Wikipedia:
“There are now 118 known elements. In this context, “known” means observed well enough, even from just a few decay products, to have been differentiated from other elements.[7][8] Most recently, the synthesis of element 118 (since named oganesson) was reported in October 2006, and the synthesis of element 117 (tennessine) was reported in April 2010.[9][10] Of these 118 elements, 94 occur naturally on Earth.”
“The remaining 24 heavier elements, not found today either on Earth or in astronomical spectra, have been produced artificially: these are all radioactive, with very short half-lives; if any atoms of these elements were present at the formation of Earth, they are extremely likely, to the point of certainty, to have already decayed, and if present in novae have been in quantities too small to have been noted.”