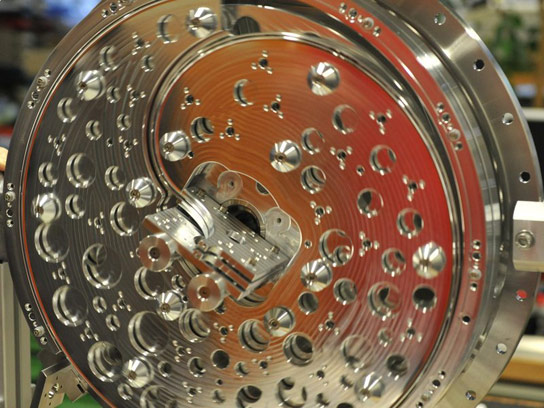
Deceleration in the centrifuge: Molecules lose speed drastically when they are guided against the centrifugal force to the center of a rotating disk. Electrodes guide the particles to the center of the centrifuge. The rotating electrodes are likewise designed as disks to ensure mechanical stability. The edges of the disks act like electrostatic guiderails for the molecules. Seen here are the inwardly bent electrodes that are used to guide the particles to the rotation axis. The electrode disks aren’t symmetric with respect to the center of the disk, so the holes in them balance them out to prevent any imbalance during rotation. Credit: MPI of Quantum Optics
A new method of using centrifugal force to decelerate particles makes it possible to produce relatively large quantities of cold molecules in a continuous flow, which could create new opportunities for chemistry and quantum information processing.
Compared with our breath, passenger planes move at a pretty leisurely pace. On average, nitrogen molecules, for example, travel at a speed of more than 1,700 kilometers (1,060 miles) per hour at room temperature, or almost one-and-a-half times the speed of sound. This means the particles are much too fast for many experiments, and also some conceivable applications. However, physicists at the Max Planck Institute of Quantum Optics in Garching have now found a rather simple way to slow down polar molecules to about 70 kilometers (43 miles) per hour. They let the molecules of various substances, such as fluoromethane, run up against the centrifugal force on a rotating disk, while being guided by electrodes. The speed of the decelerated molecules corresponds to a temperature of minus 272 degrees Celsius (minus 457 degrees Fahrenheit). The new method makes it possible to produce relatively large quantities of cold molecules in a continuous flow, which could be useful, for instance, for targeted chemical reactions of individual particles, or the processing of quantum information.
Chemical reactions are pretty uncontrolled. The reaction partners encounter each other by chance and then collide quite violently, whereupon it is not certain they will do what chemists expect them to do. Bringing them close to each other systematically and at a leisurely pace could favor some transformations that otherwise rarely occur. For this to happen, chemists need slow, and therefore cold, molecules, and they need these in large quantities. Physicists as well rely on cold molecules for many experiments, as well as for new technological applications, such as quantum information processing. For many scientists, especially in low-temperature physics, it should thus be welcome news that researchers working with Sotir Chervenkov and Gerhard Rempe at the Max Planck Institute of Quantum Optics have developed a versatile and efficient brake for polar molecules.
The Garching-based team’s decelerator slows down the particles – in their current experiments, molecules of fluoromethane, trifluoromethane, and 3,3,3-trifluoropropine – from about 700 to 70 kilometers (430 to 43 miles) per hour. Since the speed of the particles can be expressed in temperature units, this corresponds to reducing the temperature from 100 K to 1 K, or from minus 173 to minus 272 degrees Celsius (minus 279 to minus 457 degrees Fahrenheit). “Nitrogen-cooled sources supply molecules at 100 Kelvin (-173 Celsius), and we also know some good methods for further cooling molecules at 1 Kelvin,” says Sotir Chervenkov. “But there are currently no efficient methods for the range in between, and particularly none that produce a continuous flow of cold molecules.”
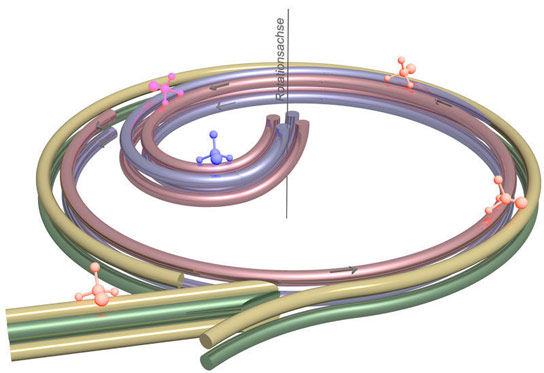
The principle of the molecular brake: Four electrodes initially guide polar molecules from the entry of the centrifuge at the lower left along the edge of a rotating disk. They are then guided in a spiral to the center of the disk. Two static electrodes at the side of the disk are shown in yellow and green, and the electrodes mounted on the disk, in violet and pink. Credit: Sotir Chervenkov/MPI of Quantum Optics
Four electrodes guide molecules to the center of the centrifuge
The Max Planck researchers rely here on an amply known force, but one that has never before been used to slow down molecules: centrifugal force. The molecular brake thus consists of a centrifuge that rotates at up to 43 revolutions per second: a 40-centimeter-in-diameter (16-inche-in-diameter) rotating disk on which the particles are guided from its periphery to its center. Four electrodes with alternating polarity spaced one millimeter apart and arranged at the apices of a square serve as guiderails imposing with their electric field a travel direction on the molecules.
Two static electrodes gird the disk brake. Through an opening in this double ring, the Max Planck physicists guide the particles into the decelerator. On the disk are likewise mounted, along almost the entire circumference, two electrodes, but not forming closed rings. Rather, the two electrodes bend in a spiral toward the center across about a quarter of the circular area.
To ensure that there are always four electrostatic guiderails keeping the molecules on track along their deceleration path, a further electrode pair accompanies the particles along the spiral coil. These electrodes are tapered and interface with the static electrode ring at a distance of just 0.2 millimeters, so that it looks as if they branched out of the ring. The molecules are thus moved smoothly onto the curved path, on which they fight against the centrifugal force and drastically lose speed until a further curve in the electrodes in the center of the disk guides them up and away from the decelerator.
Molecules would have to fly up 2,000 meters against the Earth’s gravitational field
“The deceleration is accomplished in two steps,” explains Martin Zeppenfeld, who originally devised the concept of the molecular brake. “Initially, the molecules slow down when they pass from the laboratory system to the rotating system.” This is comparable to a father running along next to his child on a rotating carousel. He moves with respect to the environment, but for the child, he’s not moving.
“Additionally, the molecules are exposed to the outwardly directed centrifugal force,” adds Martin Zeppenfeld. “On their way to the center, the particles must surmount a huge mountain, and are continuously decelerated while doing so, until they finally come almost to a standstill.” For comparison: for the particles to experience the same braking effect in the Earth’s gravitational field, they would have to fly 2,000 meters (6,600 feet) upward.
Some of the methods currently used to decelerate polar molecules use electrodes not only as guiderails, but also as the actual brake. However, with practicable field strengths, the braking effect remains low, requiring that the particles be sent repeatedly to this electrical potential mountain. This not only results in many particles being lost, but they also don’t leave the decelerator in a continuous flow, but rather in the form of particle pulses, or in other words, in batches.
Centrifuge deceleration is versatile and easy to use
“What is new about our centrifuge deceleration is its continuous operation, the large number of molecules in the resulting beams, its application versatility, and its relative ease of handling,” says Gerhard Rempe, Director at the Max Planck Institute of Quantum Optics. In principle, atoms or neutrons can also be decelerated by a centrifugal force. However, these particles aren’t polar and therefore can’t be guided through the centrifuge using an electric field.
The researchers in Garching now want to further cool the centrifuge-decelerated molecules. They aim to do this using Sisyphus cooling, which they just recently developed, and which is suitable for molecules that are already very cold. Here, an electric field decelerates the optically excited molecules. Through a combination of both methods, the researchers obtain a sufficiently dense flow of extremely cold molecules, allowing them to steer them toward one another to create specific collisions and control their chemical reactions. But the extremely cold molecules could also be accumulated to form clouds that could serve as the register of a quantum computer that is particularly fast for certain arithmetic operations. Thus, the closed cold chain for particles opens up completely new perspectives for chemistry and physics.
Reference: “Continuous Centrifuge Decelerator for Polar Molecules” by S. Chervenkov, X. Wu, J. Bayerl, A. Rohlfes, T. Gantner, M. Zeppenfeld and G. Rempe, 6 January 2014, Physical Review Letters.
DOI: 10.1103/PhysRevLett.112.013001
arXiv:1311.7119
This is a very intriguing thermodynamics for particle motion in a rotating environment for a refrigeration process. It reminds me immediately on a current Research Project at the University of Eindhoven, The Netherlands, using Vortex Tube Refrigeration, in which I am involved as a Vortex Engineering Sponsor.
Whereas in using a Vortex Tube(VT) a gas flow is split in a Hot Flow and a Cold Flow as a result of the flow dynamics and the construction details resulting in two separate Exit Ports. . . Hot Exit and a Cold Exit. . .the Centrifugal Apparatus in the SciTechDaily Link uses a single cold exit for the refrigerated gas. Moreover, although the spiral flow path of the gas (particle) flow from the peripheral inlet to the central Cold Exit is generally identical in both cases this new refrigeration process is decidedly different in at least a major aspect. In the VT process it concerns in part a simple Joule-Thomson gas expansion process for a vortex flow towards the low pressure rotation centre, providing partial thermodynamic cooling, as the gas speeds up: pressure and heat energy are transformed into kinetic energy while energy conservation applies. Additionally in the VT, due and an additional “compression-expansion” cyclic process in a recirculation flow, the gas refrigeration is enhanced, providing gas cooling to easily by 70 C in the core flow, while the peripheral flow is heated by about up to 100 C, depending on the flow fractions. The thermodynamics of this are generally easy to understand, although as a fluid dynamics process it is impossible to capture the molecular motions exactly, due to turbulence.
In contrast the cooling process in the Centrifuge is quite different, even though the Motion Pattern of the molecules as more or less individual particles. . .I assume at close to vacuum pressure level. . . is practically the same as for the gas flow in the VT. It is very clear that as a molecule enters at velocity V1 and is “guided” into the spiral path towards the central Exit by the spiral channel, two things happen: 1) The velocity V1 is split op in a Radial component Vr and a Tangential component Vt, and 2) as the molecule flows radially inwards the molecule experiences a strong retarding force due to the centripetal force caused by the tangential motion Vt, as the particle orbits at some radial position. THAT explains, principally, HOW the Molecular Brake works.
However, something happens that is NOT explained in the source text. By virtue of conservation of momentum and conservation of energy, as the molecule moves towards the centre, the tangential motion ought to INCREASE as the molecule moves radially towards the centre, but in this Centrifuge SOME PROCESS must be causing the Vt-component to be reduced as well, if the molecules are almost brought to a STOP, which by definition means that they have been refrigerated! This means that Momentum is NOT conserved, and the tangential motion is retarded in some way as well.
Perhaps the Designers want to keep THAT PART a secret :-).
Lets assume the Molecule’s Kinetic energy at Peripheral Inlet at T= 100 K is this: KE = 1/2MVt^2. . .ignoring its relativistic mass: Mr =0. As the molecule enters at speed V1=Vt then Vr =0 by definition at the Entrance, but in order to reach the centre exit the Vr must at least increase to Vr>0, but the Centripetal Force will limit its increase to an as low as possible value as the spiral flow channel on the disc spins at high speed . . . . . but what is reducing Vt???? There must be a tangential retarding force, but it can not be an electrical force, nor an magnetic force, the molecule is not charged, nor is it a magnetic mono-pole. . .
Lets bypass the Tangential Brake Mechanism that applies and look at the thermodynamics.
A molecule at T= 100K has Transactional Kinetic Energy (TKE) from velocity (Vt, +Vr) + Rotational Kinetic Energy (RKE), due to rotation of the molecule about its own axis, and Thermal Energy (TE), due to its temperature.
By virtue of Energy Conservation we have: (TKE + RKE) +TE – HT = Constant(C1).
HT is Heat Transported from the particle.
Now, the result of this is imposes the requirement that there must be a Heat Transport Process in place. As the molecule is reduced to almost zero velocity the Kinetic and Thermal Energy Sum
(TKE + RKE) +TE = HT + C1. . . . . . . .C1 is the Entry Energy at the Injection Point .
How is the energy HT + C1 – Residual Energy(RE) Transferred from the molecules to a Heat Sink?
Nothing I read explained this.
Who can answer that question?