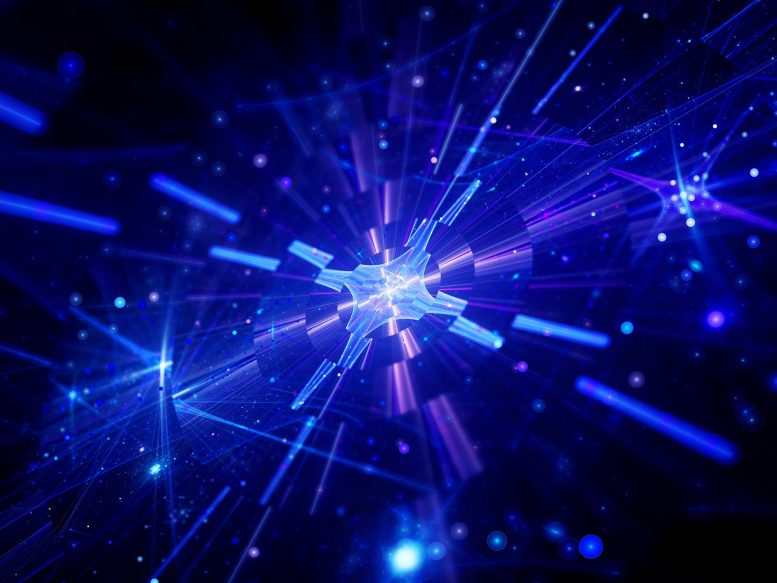
Research in synthetic dimensions is revolutionizing the field of topological photonics by enabling advanced control and manipulation of light in photonic systems, using innovations like artificial neural networks and waveguide arrays to explore new physics and optimize device applications.
Researchers developed adjustable arrays of waveguides that introduce synthetic modal dimensions, enhancing the management of light within photonic systems. This innovation has potential applications ranging from mode lasing to quantum optics and data transmission.
In the realm of physics, synthetic dimensions (SDs) have emerged as a cutting-edge research frontier, providing a means to investigate phenomena in higher-dimensional spaces beyond our conventional 3D geometry. This concept has gained substantial attention, particularly in topological photonics, due to its potential to reveal complex physics that cannot be accessed within traditional dimensions.
Researchers have proposed various theoretical frameworks to study and implement SDs, aiming at harnessing phenomena like synthetic gauge fields, quantum Hall physics, discrete solitons, and topological phase transitions in four dimensions or higher. Those proposals could lead to new fundamental understandings in physics.
One of the primary challenges in conventional 3D space is the experimental realization of complex lattice structures with specific couplings. SDs offer a solution, by providing a more accessible platform for creating intricate networks of resonators with anisotropic, long-range, or dissipative couplings.
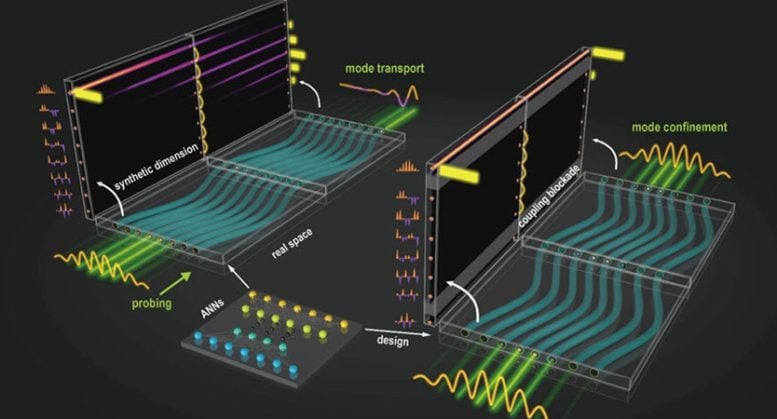
Deep learning empowers light manipulation in a synthetic dimension. Credit: Xia, Lei, et al., doi 10.1117/1.AP.6.2.026005.
This capability has already led to groundbreaking demonstrations of non-Hermitian topological winding, parity-time symmetry, and other phenomena. A variety of parameters or degrees of freedom within a system, such as frequency modes, spatial modes, and orbital angular momenta, can be used to construct SDs, promising for applications in diverse fields ranging from optical communications to topological insulator lasers.
A key goal in this field is the construction of a “utopian” network of resonators where any pair of modes can be coupled in a controlled manner. Achieving this goal necessitates precise mode manipulation within photonic systems, offering avenues for enhancing data transmission, energy harvesting efficiency, and laser array radiance.
Utilizing Artificial Neural Networks for Waveguide Design
Now, as reported in Advanced Photonics, an international team of researchers has created customizable arrays of waveguides to establish synthetic modal dimensions. This advance allows for effective control of light in a photonic system, without the need for complicated extra features like nonlinearity or non-Hermiticity. Professor Zhigang Chen of Nankai University notes, “The ability to adjust different modes of light within the system brings us a step closer to achieving ‘utopian’ networks, where all parameters of an experiment are perfectly controllable.”
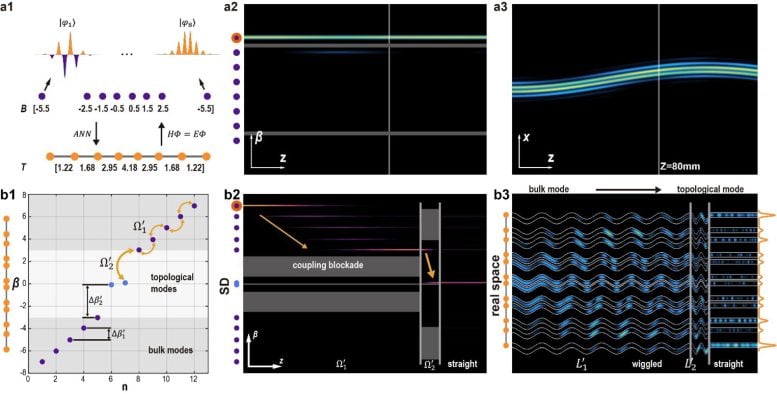
Mode confinement and topological mode morphing in a synthetic dimension designed by ANNs. (a) Illustration of the mode arrays with outlying edges of eigenvalues. (a1) Sketch of the eigenvalue array and corresponding eigenmodes. The arrangement of the coupling array in real space is calculated by ANNs. (a2) The mode evolution dynamics in SD; the orange dot in the left column indicates the excited mode. (a3) Corresponding beam propagation dynamics in real space. (b) Mode morphing in a nontrivial lattice designed by ANNs. (b1) Lattice illustration in real space and corresponding eigenvalue distribution. (b2) Mode evolution during propagation in SD; shaded zones indicate the coupling blockades in SDs in different regions. (b3) Evolution of light in real space and morphing into a topological mode; the plot on the right shows the average intensity distribution in the straight waveguide region. Credit: Xia, Lei, et al., doi 10.1117/1.AP.6.2.026005
In their work, the researchers modulate perturbations (“wiggling frequencies”) for propagations that match the differences between different modes of light. To do so, they employ artificial neural networks (ANNs) to design waveguide arrays in real space. The ANNs are trained to create waveguide setups that have exactly the desired mode patterns. These tests help reveal how light propagates and gets confined within the arrays. Finally, the researchers demonstrate the use of ANNs to design a special type of photonic lattice structure called a Su-Schrieffer-Heeger (SSH) lattice. This lattice has a specific feature enabling topological control of light throughout the system. This allows them to change the bulk mode in which light travels, showcasing the unique properties of their synthetic dimensions.
The implication of this work is substantial. By fine-tuning waveguide distances and frequencies, the researchers aim to optimize the design and fabrication of integrated photonic devices. Professor Hrvoje Buljan of University of Zagreb remarks, “Beyond photonics, this work offers a glimpse into geometrically inaccessible physics. It holds promise for applications ranging from mode lasing to quantum optics and data transmission.” Both Chen and Buljan note that the interplay of topological photonics and synthetic dimension photonics empowered by ANNs opens new possibilities for discoveries that may lead to unprecedented materials and device applications.
Reference: “Deep-learning-empowered synthetic dimension dynamics: morphing of light into topological modes” by Shiqi Xia, Sihong Lei, Daohong Song, Luigi Di Lauro, Imtiaz Alamgir, Liqin Tang, Jingjun Xu, Roberto Morandotti, Hrvoje Buljan and Zhigang Chen, 18 March 2024, Advanced Photonics.
DOI: 10.1117/1.AP.6.2.026005
Researchers have proposed various theoretical frameworks to study and implement SDs, aiming at harnessing phenomena like synthetic gauge fields, quantum Hall physics, discrete solitons, and topological phase transitions in four dimensions or higher. Those proposals could lead to new fundamental understandings in physics.
Please ask researchers to think deeply:
1. Do you really understand topological phase transitions?
2. Is universal gravitation related to topological phase transitions?
3. Are the synthetic gauge fields, quantum Hall physics, and discrete solitons related to topological phase transitions?
4. Can topological vortices form quantum gravity?
5. Is topological vortex a wave or a particle?
and so on.
Scientific research guided by correct theories can help humanity avoid detours, failures, and pomposity. Please witness the exemplary collaboration between theoretical physicists and experimentalists (https://scitechdaily.com/microscope-spacecrafts-most-precise-test-of-key-component-of-the-theory-of-general-relativity/#comment-854286). Contemporary physics has always lived in a self righteous children’s story world. Whose values have been overturned by such a comical and ridiculous reality?
Please continue performing, there will always be a large audience willing to watch these dirty and ugly things. Is this the children’s story of contemporary physics?
Misguided by the pseudo-scientific theory of Physical Review Letters (PRL), many researchers do not consider the similarities and differences between geometric shapes and physical reality in physics research, but indulge in imagination. Although scientific research can be imagined, it cannot be done recklessly.
Please ask researchers to reflect carefully.
1. Which is more suitable for explaining the physical phenomena (including universal gravitation) we observe in scientific research, topological states or imagined quantum states?
2. What is the physical reality of imagined quantum states?
3. Which is more scientific, imagined quantum states or mathematical topological states?
4. What is the correlation between mathematical graphics and real physical phenomena?
5. What is the space-time basis for the evolution of various shapes in the physical world?
and so on.
Please continue to think:
1. Is the quantum states you imagine high-dimensional matter or low dimensional matter?
2. Is topological vortex high-dimensional matter or low dimensional matter?
3. Can low dimensional matter be the understructure of high-dimensional matter?
and so on.